Global grain exports for corn and soybeans are dominated by the US and Brazil, while cereal crops derive from many countries. Asia, especially China, accounts for 43% of all grain imports.
—Rabobank, 2016
Nutrient cycling can occur in various settings and scales: on a farm, in a grassland or forest, or even globally. But the cycling of soil nutrients is intimately connected to organic matter, over half of which is carbon. So we’ll discuss both nutrients and carbon in this chapter. We use the term cycle when discussing the flow of nutrients from soil to plant to animal and back to soil, as well as global carbon and nitrogen cycles (Chapter 2). Some farmers minimize their use of nutrient supplements and try to rely more on natural soil nutrient cycles—as contrasted with purchased commercial fertilizers—to provide fertility to plants. But is it really possible to depend forever on the natural cycling of all the carbon and nutrients to maintain soil health and meet a crop’s needs? Let’s first consider what carbon and nutrient cycles are and how they differ from the other ways that carbon and nutrients move.
When carbon or nutrients move from one place to another, that is a flow, and it connects a source with a destination. There are many different types of nutrient flows that occur. When you buy fertilizers, nutrients are “flowing” onto the farm. When you buy animal feed, both nutrients and carbon are flowing onto the farm. When you sell sweet corn, apples, alfalfa hay, meat or milk, nutrients and carbon are flowing off the farm. Flows that involve products entering or leaving the farm gate are managed intentionally, whether or not you are thinking about those products in terms of nutrients or carbon. Other flows are unplanned—for example, when nitrate is lost from the soil by leaching to groundwater or when runoff waters take nutrients along with eroded topsoil to a nearby stream.
When crops are harvested and brought to the barn to feed animals, that is a nutrient flow, as is the return of animal manure to the land. Together these two flows are a cycle because nutrients and carbon return to the fields from which they came. In forests and natural grassland, the cycling of nutrients is very efficient, nearly 100%. Nutrient cycling was also efficient in the early stages of agriculture, when almost all people lived near their fields. However, in many types of agriculture, especially modern specialized farming, there is little real cycling of nutrients because there is no easy way to return the large quantity of nutrients and carbon shipped off the farm (and sometimes across continents and oceans). In addition, nutrients in crop residues don’t cycle very efficiently when the soil is without living plants for long periods; and nutrient runoff and leaching losses are much larger compared to natural systems.
Carbon and Nutrient Flows in History
Did you ever wonder why some civilizations were able to sustain agriculture for large populations while others exhausted their soils? One key component is the natural flow of carbon and nutrients. In the early days of agriculture, the productive areas were generally in low-lying locations where rivers and streams converged and flooded low-lying soils with water that contained sediments eroded from upriver soils. This annual flooding provided repeated deposits of nutrients and organic matter contained in the river’s sediments. For example, the Nile River Basin is over 1.2 million square miles in size and reaches from east-central Africa all the way to the Mediterranean. Through erosion and leaching (even under natural conditions), each area in the basin contributes small amounts of minerals (nutrients) and organic matter (carbon) that converge into the narrow downstream valley as sediment (Figure 7.1). Through the monsoonal rains in the upper basin, an annual supply of naturally fertile sediments (alluvium) was deposited on fields in the lower Nile valley and delta. This sustained a large population for several millennia. Other similar major confluence areas that were centers of ancient civilizations:
- The Indo-Gangetic Plain in parts of current day Pakistan, India, Bangladesh and Nepal, which is supplied by rivers—the Indus, Ganges and Brahmapatura—and sediment derived from the Himalayas
- The North China Plain, which is supplied by the sediment-laden Yellow River from the loess plateau in inner China
- The land between the rivers Euphrates and Tigris (Mesopotamia) in present day Syria and Iraq, which contains sediment derived from the Armenian Highlands in Turkey
- The Valley of Mexico, where ancient Lake Texcoco was fed by rivers from the surrounding fertile volcanic mountains and supported sustained wetland crop production by the Aztec civilization using raised beds (chinampas)
- Many other larger or smaller zones of alluvial deposits that were settled by tribes, including Native Americans, where they provided fertile soils and nearby sources of fish and land animals
Alluvial soils are formed from sediments deposited along the banks of streams and rivers, and in the deltas where flowing water meets the still water of a lake or ocean and sediments settle to the bottom. They tend to be very fertile because of the small-size mineral particles, organic matter (carbon) and nutrients deposited over long periods of time.
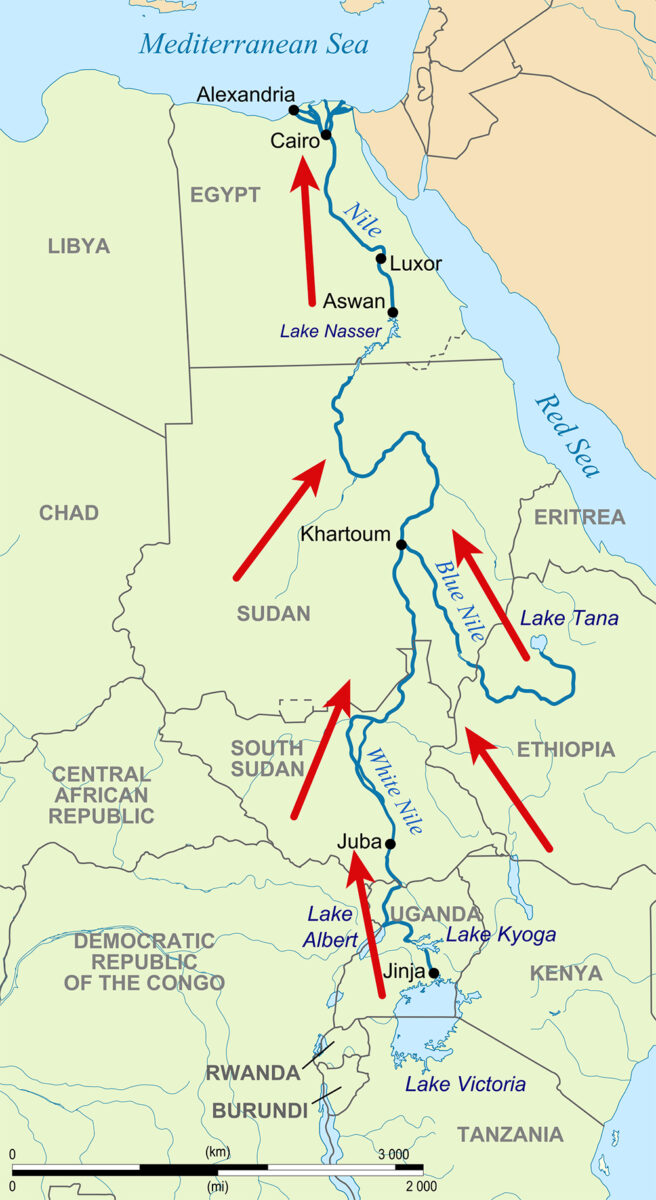
The continuous water, carbon and nutrient supplies allowed for highly productive crop production but also came with frequent flooding. In the past century, dams and levees have been constructed to reduce the impacts of flooding (and oftentimes to generate energy as well), but this means that the benefits of soil rejuvenation have ceased. Moreover, these ancient confluence zones have also become the most urbanized areas in the world, further reducing agricultural land areas. Notably, the lake in central Mexico was drained and is now occupied by the megalopolis Mexico City.
Contrasting these convergence zones in valleys and deltas, there are other regions from which the water and sediments originate, and where carbon, nutrients and water are lost. These are extensive areas away from the valleys and deltas, typically hilly or mountainous, from which resources tend to move away due to runoff, erosion and leaching. Their losses are gains for the regions downriver. In ancient times, these less productive areas were mostly used for pasturing where low-producing perennial vegetation was still valuable for extensive animal grazing, supporting small populations. Whenever such lands were taken into crop production, soils soon became exhausted from tillage, carbon and nutrient exports off the farm, and high soil erosion (Figure 7.2). Many regions thereby became unsuitable for annual crops and were converted back to pasture or to tree or vine crops (olives, grapes) that grow with low soil fertility (Figure 7.2). These farming conditions could not support large civilizations and often resulted either in declines or conquests. Notably, the low agricultural potential of the degraded hills of central Italy drove Roman conquests of the Egyptian breadbasket in the lower Nile.
Carbon and Nutrient Concentration in Soils by Human Activity
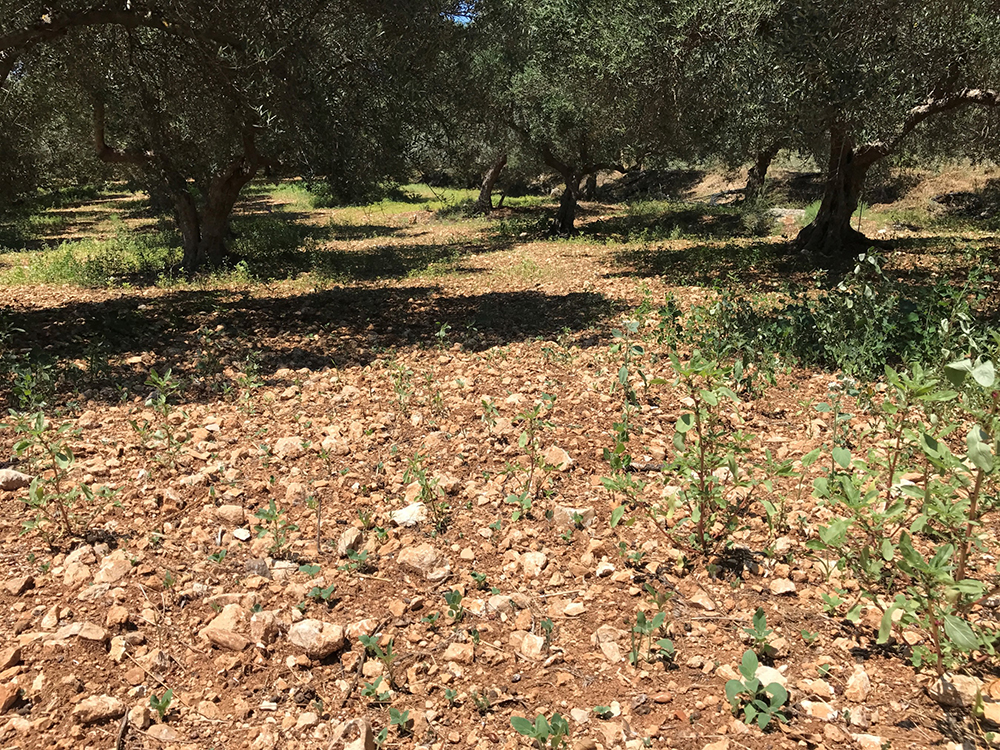
With growing global populations, more of the marginal areas were brought into production. Some were naturally productive (e.g., grassland areas in the central United States and Asia), while others were more fragile (e.g., the eastern United States). Before the availability of nutrient replenishment with artificial fertilizers, farmers sometimes built soil fertility through periodic flooding and deliberately bringing organic materials and nutrients to their crop fields, sometimes even creating soils so strongly influenced by this human activity that they’re called Anthrosols. One example is the so-called plaggen soil of northwestern Europe (Figure 7.3). These are found on low-fertility sandy soils that were not good for crop production but were suitable for pasturing. Farmers would keep areas from forest succession by cutting away the heath sod, containing plants of low nutritional value and palatability, and promoting new vegetation that could feed grazing animals, mostly sheep. But there was still a need to grow food crops. Therefore, the slices of rich sod were brought to barns where they were used as bedding for the overnighting sheep. The sod was further enriched with the sheep excrements (containing carbon and nutrients harvested from the pastures during the day), creating fertile compost that was in turn applied onto the small fields that were used to grow crops for human consumption. In this crop-pasture system, the carbon and nutrients were partly cycled on the pastures and partly flowed in a way that concentrated onto crop fields. This human ingenuity allowed for sustained animal and crop production on naturally marginal soils.
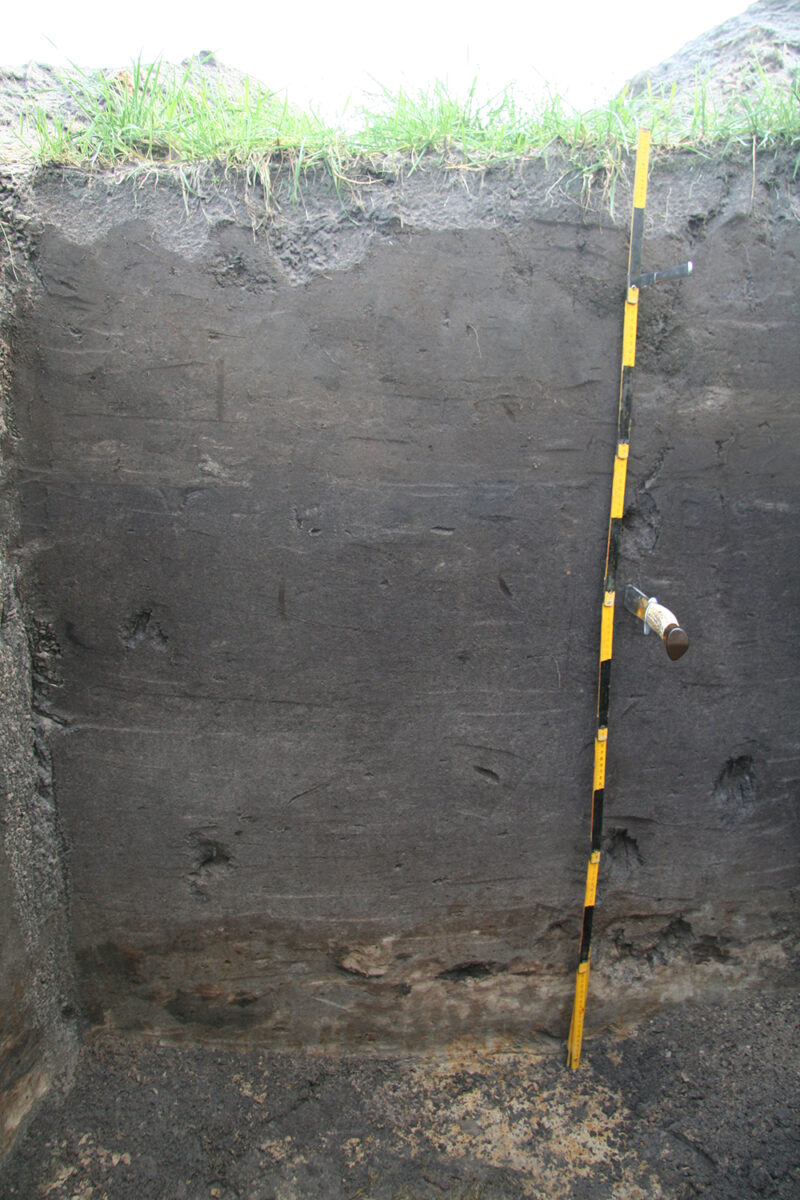
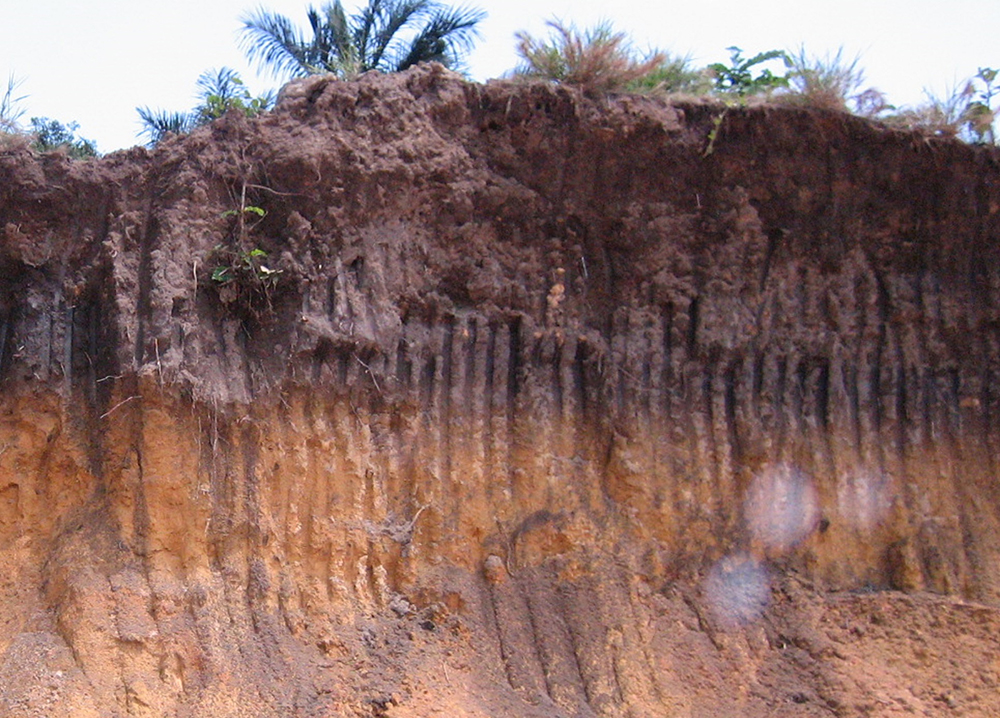
There are other examples of “dark earths” found in many settled areas around the world, notably the Amazonian Terra Preta soils that were enriched with char (as we discuss in Chapter 2). In this case, food and fuel were collected from the surrounding rainforest and were concentrated onto the soils in and around the ancient settlements. Unlike the plaggen soils, the charring of some of the organic materials created very stable organic matter that, centuries later, still keeps the soil fertile. There are many other examples of the concentration of carbon and nutrients around population settlements, including early New Englanders who used byproducts from the bountiful cod fishing industry to enhance the fertility of their croplands.
Why is this historical perspective relevant? Because today there are good opportunities to enhance soil fertility by better using organic materials. In fact, most organic farmers do just that. They bring organic materials that are often considered “wastes” onto their fields to replace the nutrients that are exported with the crops (they especially need phosphorus and potassium). Typically, this is done through compost made with tree leaves and food wastes in urban areas or through excess manure from livestock farms. Like in the past, these farmers are taking advantage of the availability of organic materials and nutrients external to their farms and are bringing them onto their fields to build soil fertility, as we discuss in detail in part 3 of this book. Different rotations and integrating cropping and livestock also offer many opportunities to “grow your own” soil organic matter and improve nutrient cycling.
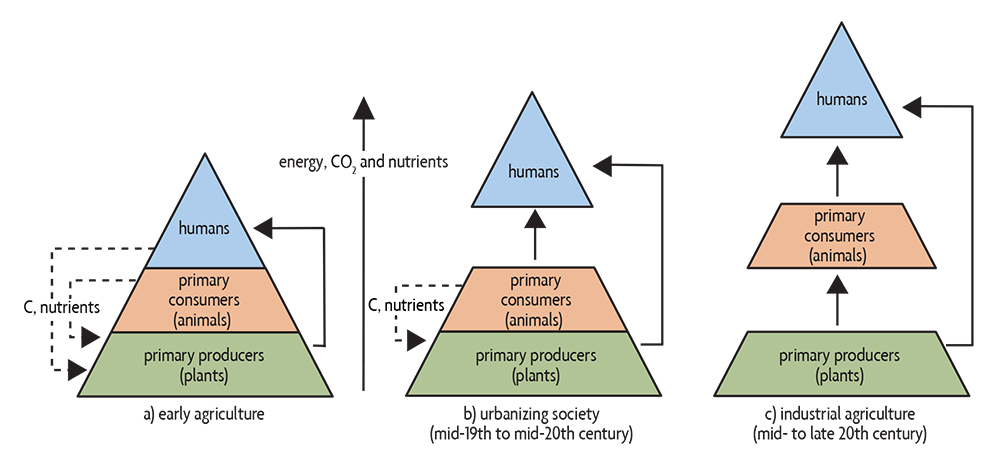
Cycling and Flows in Modern Agriculture
Older farming styles with integrated crops and animals had good cycling of carbon and nutrients. In the process of living, farm animals and humans used some of the energy and nutrients derived from plants with the remaining nutrients and carbon returned to the soil (organic residues of plants and waste materials of farm animals and humans). The first major break in this cycle occurred as cities developed and carbon and nutrients began to routinely travel with farm products to feed the growing urban populations many miles away. It is rare for the carbon and nutrients to return to the soils on which the crops and animals were originally raised (Figure 7.4b). Thus, nutrients and carbon accumulated in urban sewage and polluted waterways around the world. Even with the building of many new sewage treatment plants in the 1970s and 1980s, effluent containing nutrients still flows into waterways, and sewage sludges are not always handled in an environmentally sound manner.
The trend toward farm specialization, mostly driven by economic forces, has resulted in the second break in nutrient and carbon cycling due to the separation of animals from the land that grows their feed. With specialized large-scale animal facilities (Figure 7.4c), nutrients and carbon accumulate in manure while crop farmers purchase large quantities of inorganic fertilizers to keep their fields from becoming nutrient deficient, but they don’t usually replace all of the carbon that is lost because organic matter decomposes during the year.
Flow Patterns at Farm Scale
With relatively undisturbed forests or grasslands, the nutrients used by plants are mostly cycled back with leaf litter and the periodic dieback of roots. Carbon flows are different from the cycling that occurs when nutrients such as nitrogen, phosphorus, potassium and calcium are taken up from soil by plants, used and then returned to soil. Carbon enters the field as plants use atmospheric CO2 to carry out photosynthesis, providing the basis for all the various chemicals needed for their growth and reproduction. The portion of the plant that remains after harvest is thus added to the soil as “new” carbon in the form of organic residues; commonly this represents as much or more organic matter than was decomposed by organisms during the year.
When considering the whole farm, there are three main nutrient flow patterns, each one with implications for the long-term functioning of the farm and the environment: 1) imports of nutrients are less than exports, 2) imports are greater than exports or 3) imports are equal to exports.
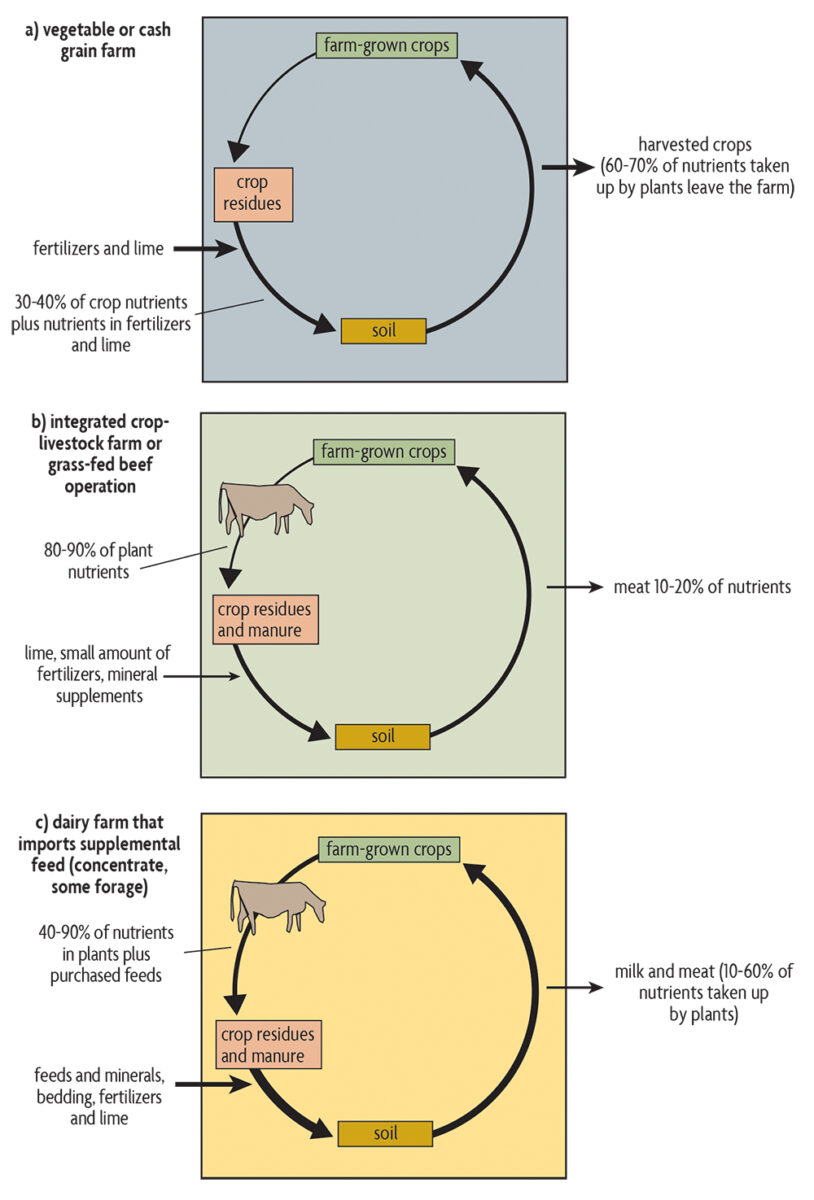
Imports are less than exports. Farms with a negative nutrient balance are “living off capital” and drawing down the supplies of nutrients from minerals and organic matter. This can continue for a while, just like a person can live off savings in a bank account until the money runs out. But at some point, the availability of one or more nutrients or organic matter (carbon) becomes so low that crop yields decrease. If this condition is not remedied, the farm becomes less and less able to produce food, and its economic condition will decline. This is clearly not a desirable situation for either the farm or the country. Unfortunately, the low productivity of much of Africa’s agricultural lands is partially caused by this pattern of nutrient and carbon flows, as increasing populations put pressure on farmers to increase land-use intensity, fertilizer prices are high for poor farmers and little attention is paid to soil organic matter. In previous times under the system of shifting cultivation, agricultural fields would have been allowed to return to forest for 20 or more years, during which time there would have been a natural replenishment of nutrients and organic matter. One of the greatest challenges of our era is to increase the fertility of the soils of Africa, both by using fertilizers and by using ecologically sound practices that increase soil health.
Imports and exports are close to balanced. From the environmental perspective and for the sake of long-term soil health, fertility should be raised to, and then maintained at, optimal levels. The best way to keep desirable levels once they are reached is to roughly balance inflows and outflows. Soil tests can be very helpful in fine-tuning a fertility program and making sure that levels are not building up too high or being drawn down too low (see Chapter 21). This can be a challenge and may not be economically possible for all farms. Farms that exclusively grow grain or vegetables have a lot of nutrients flowing onto the farm and relatively high annual carbon and nutrient exports when crops are sold (Figure 7.5a). Nutrients usually enter these farms as either commercial fertilizers or various amendments and leave the farm as plant products. Some cycling occurs as crop residues are returned to the soil and decompose. But a large outflow of carbon and nutrients is common on farms that sell considerable volumes of grains and vegetables per acre. For example, the annual export of nutrients is about 135 pounds of nitrogen, 25 pounds of phosphorus and 35 pounds of potassium per acre for corn grain and about 150 pounds of nitrogen, 20 pounds of phosphorus and 130 pounds of potassium per acre for grass hay. An acre of tomatoes or onions usually contains over 100 pounds of nitrogen, 20 pounds of phosphorus and 100 pounds of potassium. Generally, 50–60% of the carbon is harvested and exported off the farm, which in the case of corn grain amounts to about 3 tons of carbon per acre per year. But, of course, the whole point of farming in a modern society is to produce food and fiber for the non-farming public. This by necessity implies the off-farm export of carbon (sugars, starches, proteins and so on) and crop nutrients.
It should be fairly easy to balance nutrient inflows and outflows on crop farms, at least theoretically, but carbon cycling is difficult. In practice, under good management, nutrients are gradually depleted by crops until soil test levels fall too low, and then they’re raised again with fertilizers. But leftover residue (carbon-based plant material in aboveground residue and roots) from annual crops doesn’t normally replace the organic matter lost during the year of cropping. Replenishing extra soil carbon occurs only when applying organic fertilizers like manure or compost, through intensive cover cropping, or by adding perennial hay (grass/legume) crops to the rotation.
On integrated crop-livestock farms that produce their own feed, imports and exports of nutrients should be relatively small relative to the land farmed and close to balanced. Few nutrients or carbon leave the farm (they leave only as sold animals) and few are brought onto the farm (Figure 7.5b). Most of the nutrients on this type of operation complete a true cycle on the farm: They are taken up from the soil by plants, which are eaten by the animals, and most of the nutrients are then returned to the soil as manure and urine. And most of the carbon fixed by plants stays on the farm with crop residues and animal manure. A similar flow pattern with few nutrients coming onto the farm and few leaving occurs on a grass-fed beef operation that uses little to no imported feed.
It is easier to balance nutrient imports and exports on a mixed crop-livestock and grass-fed beef farms than on either a crop farm or a livestock farm that depends significantly on imported feeds. So if all the feeds are farm grown, adding an animal enterprise to a crop farm may lower the nutrient and carbon exports (Figure 7.5b).
Imports are larger than exports. Animal farms with inadequate land bases to produce all needed feed pose a different type of problem (Figure 7.5c). As animal numbers increase relative to the available cropland and pasture, larger purchases of feeds (containing nutrients) are necessary. As this occurs, there is less land available, relative to the nutrient loads, to spread manure. If the excess manure is not moved to another farm, the operation may exceed the capacity of the land to assimilate all the nutrients, and pollution of ground and surface waters occurs. For example, in a study of New York dairy farms, as animal density increased from around 1/4 of an animal unit per acre (1 AU = one 1,000-pound animal, or a number of animals that together weigh 1,000 pounds) to over 1 AU per acre, the amount of N and P remaining on farms increased greatly. When there was 1/4 AU per acre, imports and exports were pretty much in balance. But at 1 AU per acre, around 150 pounds of N and 20 pounds of P remained on the farm per acre each year.
Many dairy farms do not have the land base to grow all their needed feed and tend to emphasize growing forage crops. But the cows also need grain supplements and this situation involves additional sources of nutrients coming onto the farm. Concentrates (commonly mixtures containing corn grain and soy) and minerals usually comprise a larger source of nutrient inputs than fertilizers. In a study of 47 New York dairy farms, an average 76% of nitrogen came onto the farms as feeds and 23% as fertilizers. The percentages were pretty much the same for phosphorus (73% as feeds and 26% as fertilizers). Most of the nutrients consumed by animals end up in the manure, from 60% to over 90% of the nitrogen, phosphorus and potassium. A portion of carbon even comes onto the farm in purchased concentrate feed, and sometimes as bedding for the cows. The nutrients and carbon in manure that came from farm-grown feed sources are completing a true cycle. But the portion of nutrients in manure that originally entered the farm as purchased feeds and mineral supplements are not participating in a true cycle. These are completing a flow that might have started in a far-away farm, mine or fertilizer factory and are now being transported from the barn or feedlot to the field.
Compared with crop farms, where a high percentage of the crop grown is sold, fewer nutrients and carbon flow from dairy farms per acre and more stay on the farm, either completing a true cycle (soil to plant to animal to soil) or completing a flow (imported concentrate feed and minerals to cows to manure to soil). Because of the additional feed imports, nutrients will tend to accumulate on the farm and may eventually cause environmental harm from excess nitrogen or phosphorus. This problem of continual nutrient buildup exists for any animal farm that imports a significant percentage of its feed. The reliance on perennial forages plus imported feed and minerals, and certain types of bedding material, may increase carbon (soil organic matter) levels in the soil until they reach the soil’s saturation level. To put it another way, these farms don’t have an adequate land base to produce all their feed and therefore also have an inadequate land base on which to apply their manure at environmentally safe rates. The ultimate situations of this kind are found with animal operations that import all feeds and have a limited land base to use the manure; these have the greatest potential to accumulate high amounts of nutrients. Contract growers of poultry, with tens of thousands of chickens and few acres of land, are an example of this.
If there is enough cropland to grow most of the grain and forage needed, the result will be low amounts of imported and exported (as animal products) nutrients. It is therefore easier to rely on nutrient cycling on a mixed livestock-crop farm that produces most of its feed than on a farm growing only crops. An alternative is exchanges among neighboring farms. Since crop farms tend to have nutrient and carbon deficits, and livestock farms have excesses, transferring the excess manure or compost offers opportunities for more cycling and less environmental losses, as well as for improving soil health on the recipient farm (see Chapter 12).
The situation of imports greatly exceeding exports does not only occur on animal farms without sufficient land to grow all the needed feed. Organic vegetable farmers commonly import composted manure to supply nutrients and maintain or increase soil organic matter levels. In a survey from 2002 through 2004 of 34 organic farms from seven states in the Northeast, approximately half of the fields were found to have excessive levels of phosphorus. Other ways need to be found to add organic matter through on-farm practices such as the use of green manures, cover crops and rotations with perennial forages.
N BALANCE AS AN ENVIRONMENTAL INDICATOR
Higher nutrient imports than exports is not limited to livestock-based operations, especially with nitrogen. Most grain farms in the developed world import more nitrogen than they export through their crops, meaning the N balance is positive. As we discuss in Chapter 19, nitrogen is difficult to manage and some losses as nitrate leaching and N2O gaseous losses are unavoidable. The extent of losses is heavily dependent on how the farm manages the nitrogen through good timing and rates of applications, and through using the best product formulations and placement methods when applying commercial fertilizers. Recent research explored the use of the N balance as a simple and easily measured metric for sustainable N use. It is calculated as N inputs through nutrient additions minus N outputs through crop harvest on a seasonal basis. Optimum N balances are generally between 0 and +50 pounds per acre. If the N balance is below 0, the soil is being mined of nitrogen. If it is above 50 pounds per acre, there is excess that causes environmental damage. The 50 pounds per acre allowance reflects the fact that N use is never 100% efficient and some modest losses are often unavoidable under current practices. It is difficult for farmers to reach the optimum N balance range if they don’t carefully manage the nitrogen through the 4R practices (Chapter 18) and through the use of cover crops to catch excess nitrogen at the end of the season (Chapter 10). Better rotations that include crops that leach very low amounts of nitrate will reduce the average losses over the period of the rotation.
Distribution on the farm. A farm may aim to balance imports and exports of nutrients and carbon, but it also needs to aim for an optimum distribution onto its fields. For a portion of the year livestock farms typically concentrate their animals in barns or lots where the feed is brought in and the manure accumulates. It then needs to be returned to the fields, which in some cases may be distant from the barns/feedlots and more difficult to reach, especially with adverse weather. In the past, fields around barns received much more manure and typically had excess nutrients compared to those farther away. But with regular soil testing and good manure management planning, farms can balance nutrients and carbon for each individual field. Moreover, livestock farms that use well-planned rotational pasture systems, common in places like New Zealand, don’t have manure transportation issues and prevent nutrient concentration.
UNINTENDED NUTRIENT LOSSES
Potential problems can occur even when fertilizer imports and crop exports are more or less in balance.
This chapter considers the planned flows of nutrients and carbon purchased from off the farm as fertilizers, lime and feeds, and leaving the farm in the agricultural products sold. But what about the unintended losses of nutrients? When imports are greater than exports, nutrients accumulate on the farm and significant amounts may be lost by leaching to groundwater or in runoff waters with resulting environmental damage. Nitrogen and phosphorus are the main nutrients that flow from farms that become water pollutants.
However, even when imports and exports are approximately the same, significant unintended losses may occur. This is a concern with many crops but especially with corn production, and it becomes a substantial regional problem when a large portion of the land is devoted to this crop. With high-yielding corn, the amount of N fertilizer applied may be similar to the amount taken up by the corn: perhaps 150–160 pounds of N applied in fertilizer versus 160 pounds in the corn grain. But a lot of the N applied is tied up in organic matter or lost by leaching or denitrification. On the other hand, soil organic matter decomposes during the season and can provide a lot of nitrogen to plants. Potential pollution problems arise when the combination of soil-derived and fertilizer-derived available nitrogen greatly exceeds the crop’s need.
When corn goes through its two-month-long growth spurt, it increases rapidly in height and then fills its grain. During this phase it needs to take up large quantities of nitrogen each day, usually as nitrate. This means that high concentrations of nitrate in the soil solution are needed during this period. The problem occurs when such large amounts are supplied from fertilizer or manure applications, and when the soil is also continuing to supply even more nitrate from decomposing organic matter. In most years there’s a lot of nitrate left over after corn harvest that can leach into groundwater during the fall, winter or early spring. Rates of loss depend on the amount of precipitation during these periods but can be in the range of 30–70 pounds per acre (excess nitrate may also be converted into the greenhouse gas nitrous oxide).
The best ways to decrease soil nitrate at the end of the season and to limit nitrate losses are to 1) precisely predict the crop’s seasonal needs, accounting for all N sources including fertilizer and organic; 2) time N application close to when the crop needs it; and 3) plant a quick-establishing cover crop such as cereal rye to catch excess N. But, rotations with corn appearing less frequently would also help reduce nitrate pollution of water.
Flow Patterns at Large Scale
We have looked at carbon and nutrient balances on farms, but a larger-scale break in nutrient cycling occurs as agricultural products are shipped long distances across continents or even oceans. When you deliver a train or boat load of grain from the Midwest to the eastern United States, or even from Brazil to Asia, a lot of carbon and nutrients are flowing and don’t return. The international trade in agricultural commodities such as corn, soybeans and wheat means that significant quantities of the basic ingredient of soil health are shipped overseas. (It is worth noting because it takes so much water to produce grains: around 110 gallons (approximately 900 pounds) of water to produce one pound of corn grain or soybeans. In essence, water is also being shipped abroad embedded in the agricultural exports.)
Long-distance transportation of nutrients and carbon is central to the way the modern food system functions. On average, the food we eat has traveled about 1,300 miles from field to processor to distributor to consumer. Exporting wheat from the Pacific Northwest and soybeans from the Midwest of the United States to China involves even longer distances, as does importing apples from New Zealand to Los Angeles. The nutrients in concentrated commercial fertilizers also travel large distances from the mine or factory to distributors and then to the field, like potassium from Saskatchewan to Ohio, or phosphorus from Morocco to Germany. The specialization of the corn and soybean farms of the Midwest and the hog and chicken mega farms centralized in a few regions, such as Arkansas, the East Coast’s Delmarva Peninsula and North Carolina, has created a unique situation. This regional specialization of farms appears to make economic sense (or perhaps not?) but disrupts the nutrient and carbon cycles that maintain soil health. The nutrients from crop farms travel a long way to animal farms and are replaced with fertilizers from a completely different place. Meanwhile, the animal farms become overloaded with nutrients. The carbon exported from the crop farms never replaces the organic matter lost during the year.
Of course, the very purpose of agriculture in the modern world—the growing of food and fiber and the use of the products by people living away from the farm—results in a loss of nutrients from the soil, even under the best possible management. In addition, leaching losses of nutrients, such as calcium, magnesium and potassium, are accelerated by acidification, which occurs naturally or can be caused by the use of some fertilizers. Soil minerals, especially in the “young” soils of glaciated regions and in arid regions not subject to much leaching, may still supply lots of phosphorus, potassium, calcium, magnesium and many other nutrients even after many years of cropping. A soil with plentiful active organic matter also may supply nutrients for a long time. A mixed crop-livestock system that exports only animal products cycles the nutrients and carbon well, and it may take a long time to deplete a rich soil because few nutrients are exported with those products.
But for crop farms, especially in humid regions, the depletion occurs more rapidly because more nutrients are exported per acre each year. Eventually a continually cropped soil becomes nutrient depleted, and sooner or later you will need to apply some phosphorus or potassium. Nitrogen is the only nutrient you can “produce” on the farm: legumes and their bacteria working together can remove nitrogen gas from the atmosphere and change it into forms that plants can use.
The issue eventually becomes not whether nutrients will be imported onto the farm but rather what source of nutrients you should use. Will the nutrients brought onto the farm be commercial fertilizers; traditional amendments (limestone); biologically fixed nitrogen; imported feeds or minerals for livestock; organic materials such as manures, composts, and sludges; or some combination of sources? Some nutrient sources are nutrient dense and therefore efficiently transported and applied, like inorganic fertilizers. But they don’t provide the benefits of carbon, which is critical to the biological processes in the soil. Organic sources provide the benefits of both nutrients and carbon, but the nutrients are in low concentrations and expensive transport effectively restricts their application to nearby locations.
Finally, a few words about a positive aspect of large-scale nutrient flows. National and international food trade occasionally provides benefits by reducing the effects of regional micronutrient deficiencies in the importing country. For example, selenium is a trace element that most humans and animals acquire from the soil through their diets. European selenium intake is enhanced by importing wheat grown on U.S. soils, which are naturally higher in the nutrient.
GLOBAL GRAIN TRADE AND NUTRIENT-CARBON FLOWS
Several grain and oil crops are heavily traded around the world, including wheat, corn, soybeans, rice and oilseeds. They greatly impact the global flow of nutrients and carbon, the basic ingredients for healthy soil. The most prominent transfers involve corn and soybeans shipped from the Americas to East Asia, Europe and the Middle East. Why is that?
The import of these grain crops is heavily driven by higher demand for animal protein and edible oils as a result of rising living standards and diversifying diets. Growing these crops also requires a large land base that many countries do not have. Japan and Korea are populous but also quite mountainous. The Middle East and Mexico are dry, and Europe has more animals than it can feed from its own land. China is by far the largest grain importer, especially of soybeans, because of agronomic constraints and domestic policies that prioritize growing cereal crops that meet basic food security. A large portion of the crops that feed its animals are therefore imported. (China raises about half the world’s pigs.)
The Americas have a large agricultural land base and are the primary exporter of those grains, with the United States, Brazil and Argentina accounting for almost 90% of the soybean and 75% of the global corn exports that are mostly used to raise animals in other parts of the world (Figure 7.6). These countries also have policies that promote grain production and exports. U.S. grain areas have been fairly stable over the past decades, but the South American countries have met the higher global demand by putting extensive areas of grassland, savannah and even rainforest into crop production.
Wheat and rice are different, not only because they are mostly consumed directly by humans. Wheat export is more balanced among countries with Russia (20%), Canada (14%), the United States (13%), France (10%), Australia (8%) and Ukraine (7%) being the top exporters. Rice tends to be grown more for domestic consumption and therefore not exported as much, but India (30%) and Thailand (23%) are the main international suppliers.
With the large transfer of grains, and associated carbon and nutrients, from one region to another, deficiencies are created with the exporters and excesses with the importers. Evidence is emerging that the breadbasket soils in the Americas are becoming less healthy and have lost organic matter. The importing countries have many livestock farms that accumulate nutrients and have water pollution concerns. Hypoxia (dead zones) is an increasing problem in the seas around Japan, Korea, China and northern Europe.
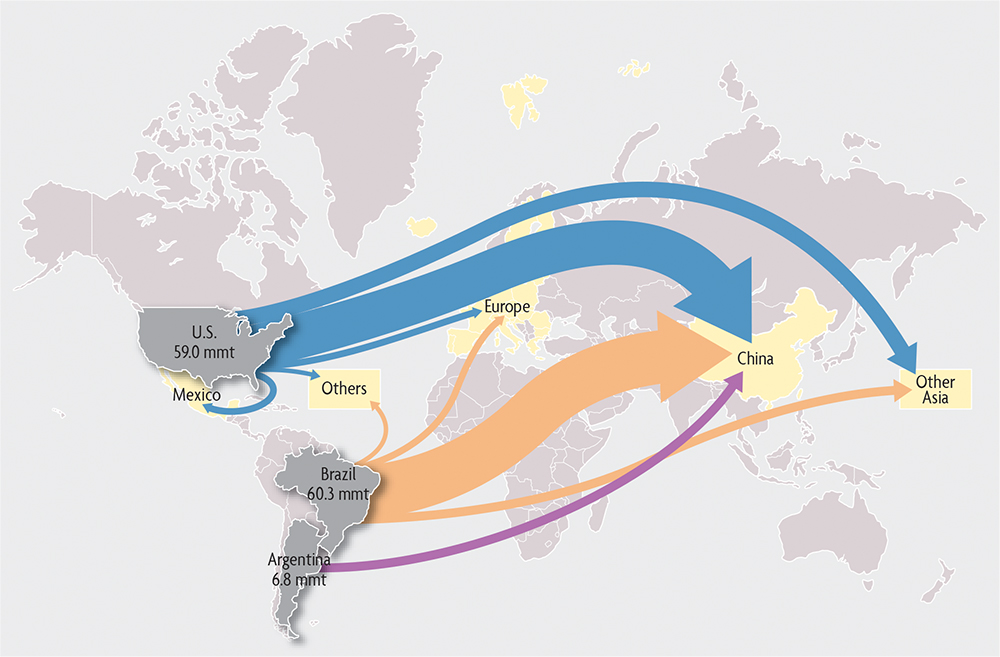
Chapter 7 Summary
The cycling and flow of nutrients and carbon have been critical to agriculture since its beginning. Soils along water courses amassed sediments and nutrients from upriver regions and allowed for sustained productivity. Most other areas experienced losses associated with soil degradation. There is good nutrient cycling when crop residues or animal manures are returned to the soil and feed the subsequent crops. However, there are potentially large flows of nutrients and carbon into and out of farms, and we are concerned about cases where the flows are very unbalanced. The inflow occurs as commercial and organic fertilizers or animal feeds are imported onto the farm. Managed exports are mainly in the form of crops and animal products. In general, larger amounts of nutrients are exported off the farm in vegetation (grains, forages, vegetables, etc.) than in animal products. This happens because a high percent of the nutrients and carbon in the feeds pass through the animal and stay on the farm as manure, and relatively few are exported in the form of milk, meat, wool, etc., compared to farms exporting their crops.
Our modern agricultural system has intensified problems with nutrient flows. Negative balances of nutrient and carbon flows are of great concern because the soil degrades as their levels decline. On the other hand, when nutrient balances are highly positive and build up on the farm, they tend to be more readily lost to the environment. Cash grain farm regions like the Midwestern United States and Brazil export a lot of nutrients and carbon, and also lose nutrients to the environment due to inefficient fertilizer use to replace the lost nutrients. Regions that import a lot of grain to feed livestock create excessive levels of carbon and nutrients. All these farms negatively impact water quality, marine ecosystems and greenhouse gas emissions.
Chapter 7 Sources
Anderson, B.H. and F. Magdoff. 2000. Dairy farm characteristics and managed flows of phosphorus. American Journal of Alternative Agriculture 15: 19–25.
Gale, F., C. Valdes and M. Ash. 2019. Interdependence of China, United States and Brazil in soybean trade. USDA ERS Report OCS-19F-01.
Harrison, E., J. Bonhotal and M. Schwarz. 2008. Using Manure Solids as Bedding. Report prepared by the Cornell Waste Management Institute (Ithaca, NY) for the New York State Energy Research and Development Authority.
Kabir, Z. 2017. Rethinking the Nutrient Management Paradigm for Soil Health, Conservation webinar, USDA NRCS, http://www.conservationwebinars.net/webinars/rethinking-the-nutrient-management-paradigm-for-soil-health.
Magdoff, F., L. Lanyon and W. Liebhardt. 1997. Nutrient cycling, transformations, and flows: Implications for a more sustainable agriculture. Advances in Agronomy 60: 1–73.
Magdoff, F., L. Lanyon and W. Liebhardt. 1998. Sustainable Nutrient Management: A Role for Everyone. Northeast Region Sustainable Agriculture Research and Education Program: Burlington, VT.
Morris, T.F. 2004. Survey of the nutrient status of organic vegetable farms. SARE project database, https://projects.sare.org/project-reports/lne01-144/.
Rabobank. 2016. Grow with the flow. Rabobank Industry Note #541. Available at Rabobank.com.Rasmussen, C.N., Q.M. Ketterings, G. Albrecht, L. Chase and K.J. Czymmek. 2006. Mass nutrient balances: A management tool for New York dairy and livestock farms. In Silage for Dairy Farms: Growing, Harvesting, Storing, and Feeding, pp. 396–414. NRAES Conference, Harrisburg, PA, January 23–25.