A lasting injury is done by ploughing land too wet.
—S.L. Dana, 1842
We’ve already discussed the benefits of cover crops, rotations, reduced tillage and organic matter additions for improving soil structure. However, these practices still may not prevent compacted soils unless specific steps are taken to reduce the impact of heavy loads from field equipment and inappropriately timed field operations. The causes of compaction were discussed in Chapter 6, and in this chapter we discuss strategies to prevent and lessen soil compaction. If measures to loosen a severely compacted soil are not taken, yield losses may be significant. One study in the Upper Midwest estimated a median 21% yield reduction for corn and soybeans on lands that experienced deep wheel traffic compaction at harvest. Urban areas also often experience big problems with soil compaction, which we discuss separately in Chapter 22.
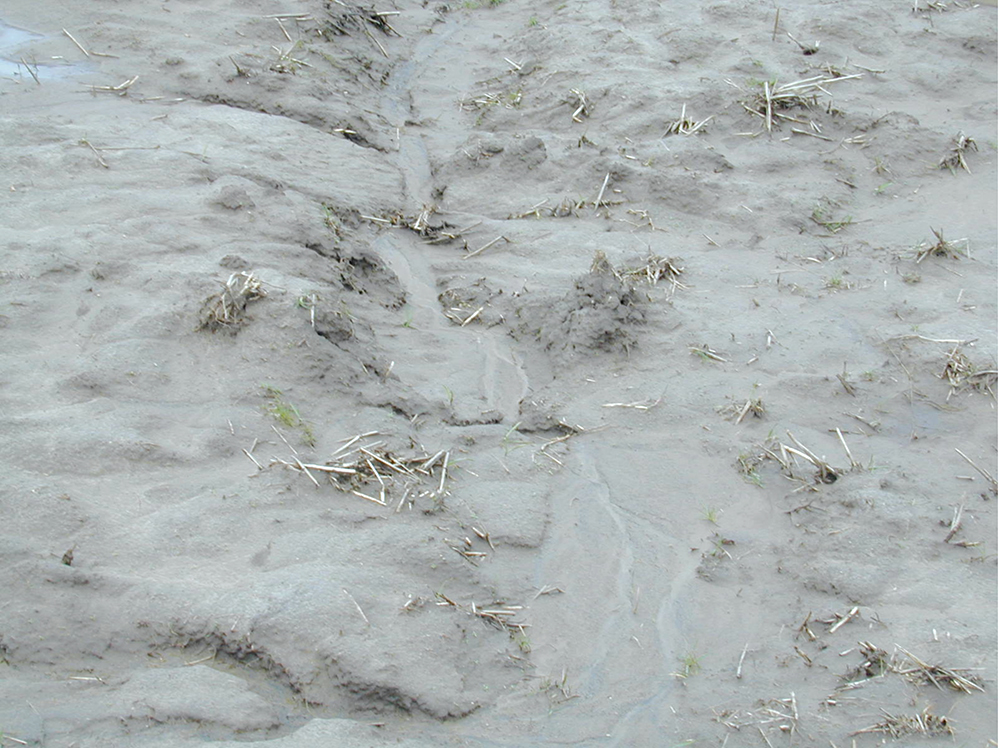
Diagnosing Different Types of Compaction
The first step is to decide whether compaction is a problem and which type is affecting your soils. The symptoms, as well as remedies and preventive measures, are summarized in Table 15.1.
Surface Sealing and Crusting
This type of compaction occurs at the immediate soil surface when the soil is exposed. It may be seen in the early growing season, especially with clean-tilled soil, and in the fall and spring after a summer crop (Figure 15.1). Certain soil types, such as sandy loams and silt loams, are particularly susceptible. Their aggregates usually aren’t very stable, and once broken down, the small particles fill in the pore space between the larger particles, making very dense crusts.
Table 15.1 Types of Compaction and Their Remedies | ||
---|---|---|
Compaction type | Indications | Remedies/prevention |
Surface seal or crust | Breakdown of surface aggregates and sealing of surface Poor seedling emergence Accelerated runoff and erosion | Eliminate tillage or reduce tillage intensity Maximize surface cover: leave residues on surface, grow cover crops Add organic matter |
Surface layer | Deep wheel tracks Prolonged saturation or standing water Poor root growth and more disease symptoms Hard to dig and resistant to penetrometer Cloddy after tillage | Use zone builders or strip tillers to break compaction but minimize soil disturbance Use cover crops or rotation crops that can break up compacted soils Add organic matter Use better load distribution with equipment Use controlled traffic Don’t travel on soils that are wet Improve soil drainage |
Subsoil | Roots can’t penetrate subsoil Resistant to penetrometer at greater depths | Don’t travel on soils that are wet Improve soil drainage Till deeply with a zone builder or strip tiller Use cover crops or rotation crops that penetrate compact subsoils Use better load distribution Use controlled traffic Don’t use wheels in open furrows |
The impact of surface sealing and crusting is most damaging when heavy rains occur between planting and seedling emergence, when the soil is most susceptible to raindrop impact. Keep in mind that this may not happen every year. The hard surface crust may delay seedling emergence and growth until the crust mellows with the next rains. If such follow up showers do not occur, the crop may be set back. Crusting and sealing of the soil surface also reduce water infiltration capacity, which can increase runoff and erosion and lessen the amount of available water for crops.
Surface Layer Compaction
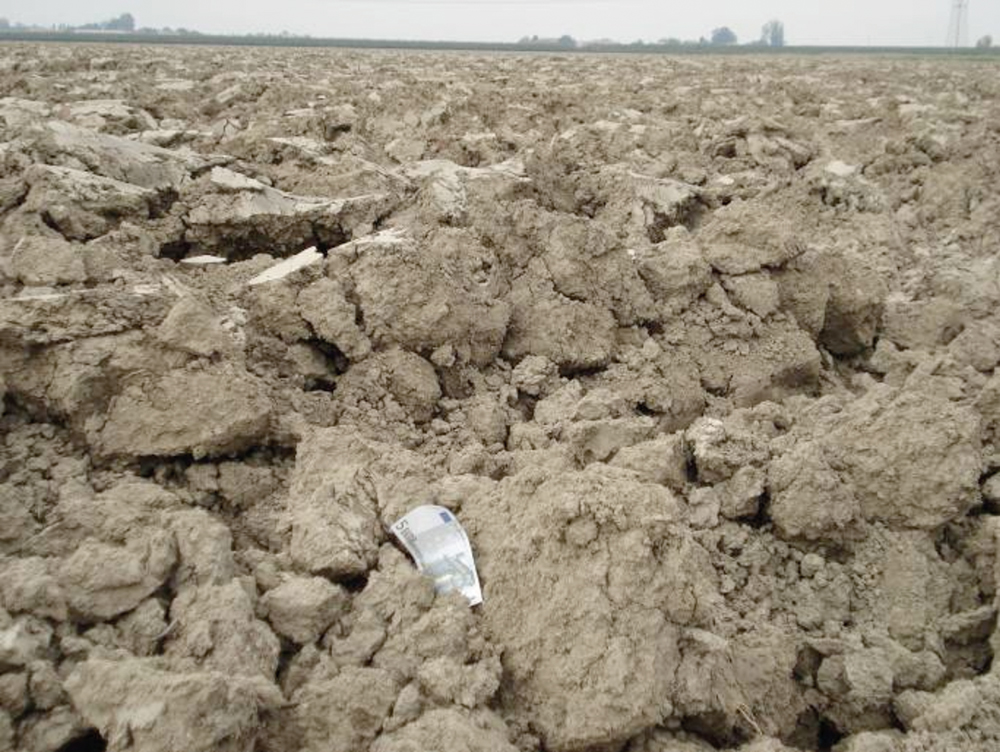
Compaction of the layer immediately below the surface can often be observed in the field through deep wheel tracks, extended periods of saturation, or even standing water following rain or irrigation. Compacted surface layers also tend to be extremely cloddy when tilled (Figure 15.2). A field penetrometer, which we discuss in greater detail in Chapter 23, is an excellent tool to assess soil compaction (you can also push a simple wire flag into the soil). Digging with a shovel allows for direct visual evaluation of soil structure and rooting, as well as of the overall quality of the soil. This is best done when the crop is in an early stage of development but after the rooting system has had a chance to establish. Well-structured soil shows good aggregation, is easy to dig and will fall apart into granules when you throw a shovelful on the ground. If you find a dense rooting system with many fine roots that protrude well into the soil, you probably do not have a compaction problem. Conversely, roots in a compacted surface layer are usually stubby and have few root hairs (Figure 15.3). They often follow crooked paths as they try to find zones of weakness in the soil. Compare the difference between soil and roots in wheel tracks and nearby areas to observe compaction effects on soil structure and plant growth behavior. Note that recently plowed soils may give a false impression of compaction: they are initially loose but will likely compact later in the season. No-tilled soils generally are firmer but have stronger structure and contain large pores from worm activity.
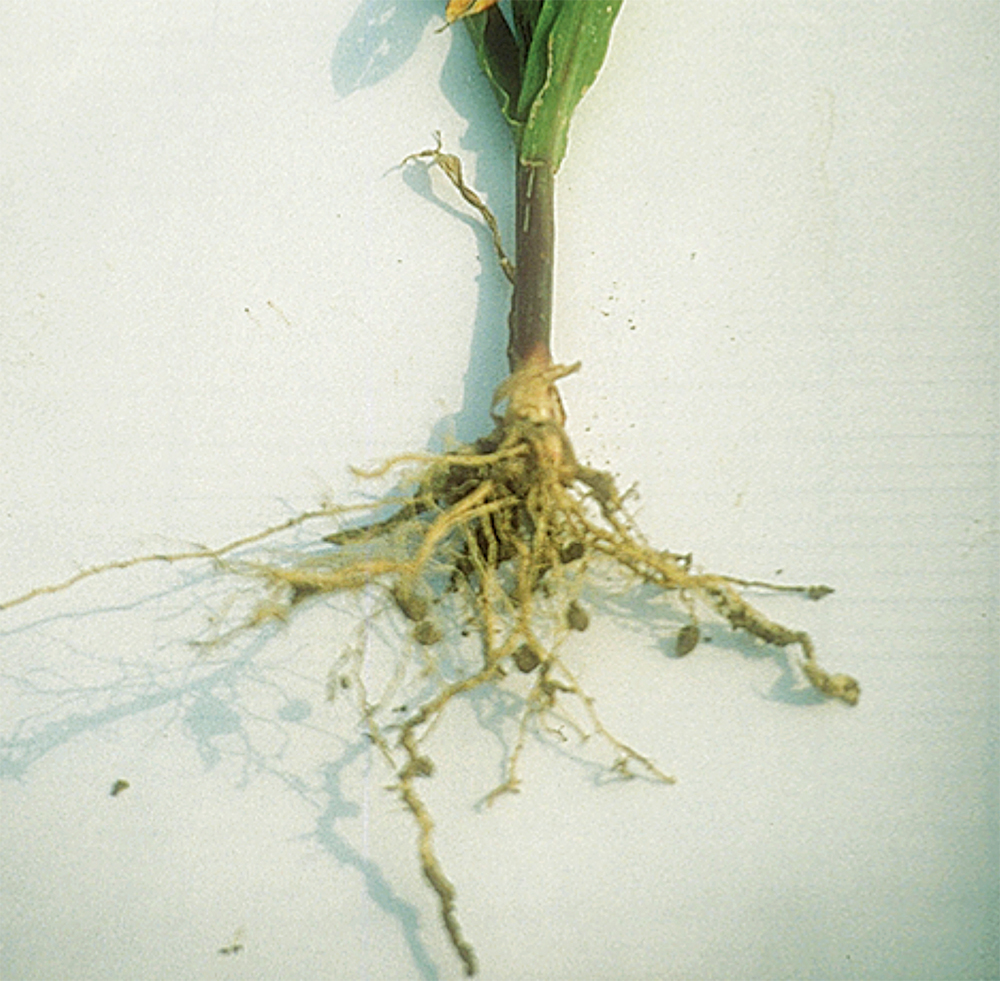
Compaction may also be recognized by observing crop growth. A poorly structured surface layer will settle into a dense mass after heavy rains, leaving few large pores for air exchange. If soil wetness persists, anaerobic conditions may occur, causing reduced growth and high denitrification losses (exhibited by leaf yellowing), especially in areas that have drainage problems. In addition, these soils may “hard set” if heavy rains are followed by a drying period. Crops in their early growth stages are very susceptible to these problems (because roots are still shallow), and the plants may go through a noticeable period of stunted growth on compacted soils.
Reduced growth caused by compaction also affects the crop’s ability to fight or compete with pathogens, insects and weeds. These pest problems may become more apparent, therefore, simply because the crop is weakened. For example, during wet periods, dense, poorly aerated soils are more susceptible to infestations of fungal root diseases such as Phytophthora, Sclerotinia, Fusarium, Pythium, Rhizoctonia and Thieviopsis and plant-parasitic nematodes such as northern root-knot. These problems can be identified by observing washed roots. Healthy roots are light colored, while diseased roots are black or show lesions. In many cases, soil compaction is combined with poor sanitary practices and lack of rotations, creating a dependency on heavy chemical inputs.
Subsoil Compaction
Subsoil compaction is difficult to diagnose because the lower soil layers are not visible from the surface. The easiest way to assess compaction in deeper soil layers is to use a penetrometer, which should be done when the soil is field-moist (not too wet, not too dry). It is surprising how often you find the tool hitting much higher resistance once it reaches the bottom of the plow layer—typically down 6–8 inches—even if it has not actually been tilled for awhile. Rooting behavior below the surface layer is also a good indicator for subsoil compaction, assuming you are willing to expend some effort digging to that depth. Roots are almost completely absent from the subsoil below severe plow pans and often move horizontally above the pan (see Figure 6.8). Keep in mind, however, that naturally shallow-rooted crops, such as spinach and some grasses, may not necessarily experience problems from subsoil compaction.
Some soils are naturally susceptible to the formation of dense subsoils when they become intensively cropped. When soil aggregates become weaker from loss of the organic matter, silt and clay particles can wash down and settle in the subsoil pores, thereby creating a dense layer. This is especially a concern with soils that contain about equal amounts of sand, silt and clay, and where the clay minerals are of the non-swelling 1:1 type. Also, tropical oxisols have naturally high clay contents with very strong aggregates, but when they are limed, the raised pH causes the clay particles to disperse and wash into pores in the lower soil.
CROPS THAT ARE HARD ON SOILS
Some crops are particularly hard on soils:
- Root and tuber crops like potatoes require intensive tillage and a lot of disturbance at harvest. They also return low rates of residue to the soil.
- Silage corn and soybeans return low rates of residue.
- Many vegetable crops require a timely harvest, so field traffic occurs even when the soils are too wet.
Special care is needed to counter the negative effects of such crops. Counter measures may include selecting soil-improving crops to fill out the rotation, using cover crops extensively, using controlled traffic, and adding extra organic materials such as manures and composts. Some potato farmers in New York and Maine are known to rotate fields with dairy farmers who convert them into soil-building alfalfa and grass. In an 11-year experiment in Vermont with continuous corn silage on a clay soil, we found that applications of dairy manure were critical to maintaining good soil structure. Applications of 0, 10, 20 and 30 tons (wet weight) of dairy manure per acre (1 ton per acre equals 2.2 metric tons per hectare) each year of the experiment resulted in pore spaces of 44%, 45%, 47% and 50% of the soil volume, respectively.
Relieving and Preventing Compaction
Preventing or reducing soil compaction generally requires a comprehensive, long-term approach to addressing soil health issues and rarely gives immediate results. Compaction on any particular field may have multiple causes, and the solutions are often dependent on the soil type, climate and cropping system. With some exceptions, let’s go over some general principles of how to solve these problems.
Reducing surface sealing and crusting. Crusting is a symptom of the breakdown of soil aggregates that occurs especially with intensively and clean-tilled soils. As a short-term solution, farmers sometimes use tools like rotary hoes to break up the crust. The best long-term approach is to reduce tillage intensity (or eliminate tillage altogether), leave residue or mulch on the surface, plant cover crops, and improve aggregate stability with organic matter additions. Even residue covers as low as 30% of the soil surface area will greatly reduce crusting and provide important pathways for water entry. A good, heavy duty conservation planter—with rugged coulter blades for in-row soil loosening, tine wheels to remove surface residue from the row, and accurate seed placement—can be a highly effective implement because it can successfully establish crops without intensive tillage (see Chapter 16). Reducing tillage and maintaining significant amounts of surface residues not only prevent crusting but also rebuild the soil by increasing aggregation. Soils with very low aggregate stability, especially those high in sodium, may sometimes benefit from surface applications of gypsum (calcium sulfate). Aggregation is promoted by the added calcium and the effect of the greater salt concentration in the soil water as the gypsum dissolves.
Reducing surface layer compaction through proper use of tillage. Tillage can either cause or lessen problems with soil compaction. Repeated intensive tillage reduces soil aggregation and compacts the soil over the long term, causes erosion and loss of topsoil, and may bring about the formation of plow pans. On the other hand, tillage can relieve compaction by loosening the soil and creating pathways for air and water movement and root growth. This relief, however, as effective as it may be, is temporary and would probably need to be repeated in the following growing seasons if poor soil management and traffic patterns are continued. Farmers frequently use more intense tillage to offset the problems of cloddiness associated with compaction of the plow layer. But this puts them in a downward cycle and it should be avoided.
ORGANIC MATTER HELPS SOILS RESIST COMPACTION
Organic matter in all its forms—including living roots, soil organisms, fragments of crop residue and dead organisms, and the “very dead”—helps to resist soil compaction in a number of ways. Using cover crops, leaving residues on the surface and increasing soil organic matter levels are indicated in Table 15.1 as remedies for all forms of compaction. The ways in which organic matter help:
- Residue on the surface helps dissipate the compacting force of equipment.
- Soil organic matter is less dense than mineral particles.
- Remnants of plant residue and soil organisms (the dead) help stabilize aggregates.
- Roots and large soil organisms (the living) create channels and other spaces for air and water movement and storage. Roots and mycorrhizal fungi secrete sticky substances that promote formation of stable aggregates and help bind smaller aggregates into larger ones. Some cover crops are able to break through compacted layers, allowing roots of the following commercial crops to better penetrate the soil. And the extensive root systems of perennial forage crops in a rotation, undisturbed by tillage, work to improve soil structure.
The long-term solution to these problems is to eliminate or significantly reduce tillage and to better manage soil organic matter (see below), but not necessarily to stop tillage altogether right away. Compacted soils frequently become “addicted” to tillage, and converting to no-till “cold turkey” may result in failure. Practices that perform some soil loosening with minor disturbance at the soil surface may help in the transition from a tilled to an untilled management system. Aerators (Figure 15.4) have rotating tines that provide shallow compaction relief in dense surface layers but do minimal tillage damage and are especially useful when aeration is of concern. They are also used to incorporate manure with minimal tillage damage. Strip tillage (6–8 inches deep) employs narrow shanks that disturb the soil only where future plant rows will be located (Figure 15.4). It is especially effective at promoting root proliferation. This practice is a good transition to more pure no-till, but many farmers find strip tillage a good long-term strategy that gets them similar benefits to no-till with fewer concerns about compaction. Yeoman’s plows achieve similar results with virtually no surface disturbance.
Another approach may be to combine organic matter additions (compost, manure, etc.) with reduced tillage intensity and a planter that ensures good seed placement with minimal secondary tillage. Such a soil management system builds organic matter over the long term. In general, the benefits of no-till take 2–5 years to be realized, but this timeline can be accelerated with the use of cover crops.
Relieving subsoil compaction through deep tillage. Deeper tillage may be beneficial on soils that have developed a plow pan or other deep compacted layers. Simply shattering this pan allows for deeper root exploration. To be effective, deep tillage needs to be performed when the entire depth of tillage is sufficiently dry and in the friable state, otherwise it causes smearing of the soil.
Subsoiling relates to a range of methods to alleviate compaction below the 6- to 8-inch depths of normal tillage and is often done with heavy duty rippers (Figure 15.5) and large tractors. Subsoiling is often erroneously seen as a cure for all types of soil compaction, but it does relatively little to address surface layer compaction that is caused by aggregate breakdown. Subsoiling is a rather costly and energy consuming practice, and its use is difficult to justify on a regular basis. Also, large rippers generally cause more soil disturbance than needed, and practices such as zone building and deep strip tillage (Figure 15.4) are better ways to loosen the soil below the plow layer. They have narrow shanks that disturb the soil less and leave crop residues on the surface (Figure 15.5). Deep tillage tends to be more effective on coarse-textured soils (sands, gravels), as crops on those soils respond better to deeper rooting. In fine-textured soils, the entire subsoil often has high strength values, so the effects of deep tillage are less beneficial. In some cases it may even be harmful for those soils, especially if the deep tillage was performed when the subsoil was wet and caused smearing, which may generate drainage problems. After performing deep tillage, it is important to prevent future re-compaction of the soil by keeping heavy loads off the field and not tilling the soil when inappropriate soil moisture conditions exist, because otherwise the benefits are short lived. Soils that are naturally susceptible to subsoil compaction due to the washing down of fine particles may need repeated deep tillage.
Lessening and preventing soil compaction are both important to improving soil health. The specific approaches should meet the following criteria:
- They should be selected based on where the compaction problem occurs (subsoil, surface layer, or at the very surface).
- They must fit the soil and cropping system and their physical and economic realities.
- They should be influenced by other management choices, such as the tillage system and use of organic matter amendments.
Better attention to working the soil and field traffic. Compaction of the plow layer or subsoil is often the result of working the soil or running equipment when it is too wet (Figure 15.6). Avoiding this may require equipment modifications and different timing of field operations. The first step is to evaluate all traffic and practices that occur on a field during the year and determine which operations are likely to be most damaging. The main criteria should be:
- the soil moisture conditions when the traffic occurs, and
- the relative compaction effects of various types of field traffic (mainly defined by equipment weight and load distribution).
For example, with a late-planted crop, soil moisture conditions during tillage and planting may generally be dry, and minimal compaction damage occurs. Likewise, mid-season cultivations usually do little damage because conditions are usually dry and the equipment tends to be light. However, if the crop is harvested under wet conditions, heavy harvesting equipment and uncontrolled traffic by trucks that transport the crop off the field will do considerable compaction damage. In this scenario, emphasis should be placed on improving the harvesting operations. In another scenario, a high-plasticity clay loam soil is often spring plowed when still too wet. Much of the compaction damage may occur at that time, and alternative approaches to tillage and timing should be a priority.
Better load distribution. Using improved designs of field equipment may help reduce compaction problems by better distributing vehicle loads. The best example is the use of tracks (Figure 15.7), which greatly reduce the potential for subsoil compaction. But beware! Tracked vehicles may provide a temptation to traffic the land when the soil is still too wet. Tracked vehicles have better flotation and traction, but they can still cause compaction damage, especially through smearing under the tracks. Surface layer compaction may also be reduced by using flotation tires and lowering the air pressure in the tires. A rule of thumb: Cutting tire inflation pressure in half doubles the size of the tire footprint to carry an equivalent equipment load and cuts the contact pressure on the soil in half.
The use of multiple axles reduces the load carried by individual wheels and tires. Even though the soil receives more tire passes by having a larger number of tires, the resulting compaction is still reduced (most compaction occurs from the first tire and subsequent tires result in little additional damage). Using large, wide tires with low inflation pressures also helps reduce potential soil compaction by distributing the equipment load over a larger soil surface area. Use of dual wheels similarly reduces compaction by increasing the footprint, although this load distribution is less effective for reducing subsoil compaction because the pressure cones from adjacent tires (see Figure 6.12) merge at shallow depths. Dual wheels are very effective at increasing traction but, again, pose a danger because of the temptation (and ability) to do fieldwork under relatively wet conditions. Duals are not recommended on tractors for performing seeding and planting operations because of the larger footprint (see also discussion on controlled traffic below). Improved soil drainage. Fields that do not drain in a timely manner often have more severe compaction problems. Wet conditions persist in these fields, and traffic or tillage operations often are done when the soil is too wet. Sometimes, the fields are plowed when the bottom of the plow layer is still too wet, causing smearing and plow pans. Improving drainage may go a long way toward preventing and reducing compaction problems on poorly drained soils. Subsurface (tile) drainage improves timeliness of field operations, helps dry the subsoil and, thereby, reduces compaction in deeper layers. On heavy clay soils where the need for close drain spacing is very expensive, surface shaping and mole drains are effective methods. Drainage is discussed in more detail in Chapter 17.
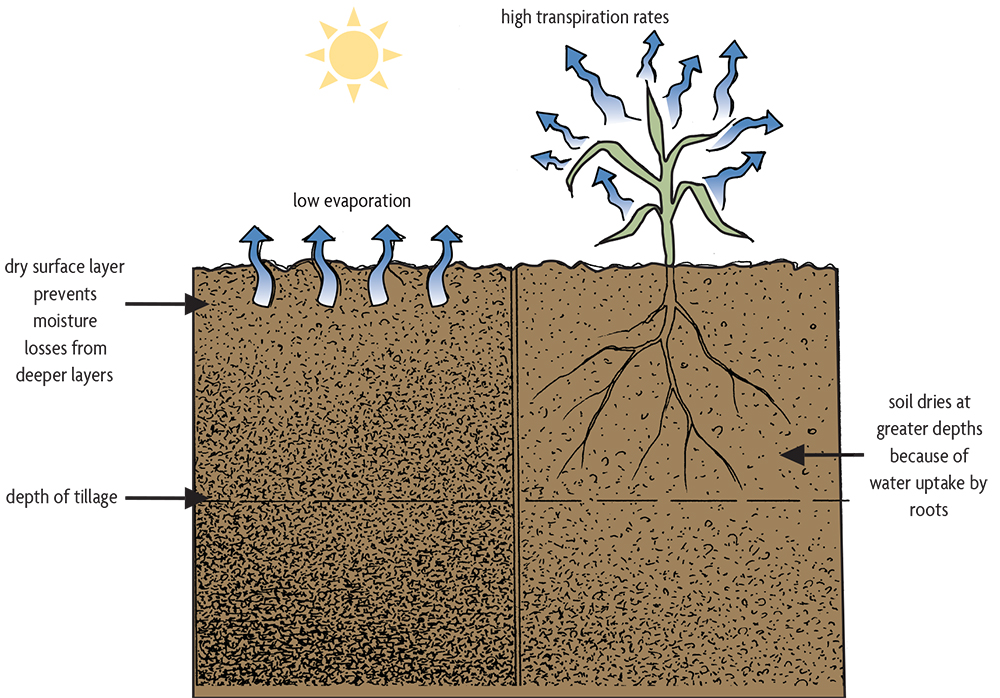
Clay soils often pose an additional challenge with respect to drainage and compaction because they remain in the plastic state for extended periods after wet conditions. Once the upper inch of the soil surface dries out, it becomes a barrier that greatly reduces further evaporation losses. This is often referred to as self-mulching. This barrier keeps the underlying soil in a plastic state, preventing it from being worked or trafficked without causing excessive smearing and compaction damage. For this reason, farmers often fall-till clay soils. A better approach, however, might be to use winter cover crops to dry the soil in the spring. When a crop like cereal rye grows rapidly in the spring, the roots effectively pump water from layers below the soil surface and allow the soil to transition from the plastic to the friable state (Figure 15.8). Because these soils have high moisture-holding capacity, there is normally little concern about cover crops depleting water for the following crop.
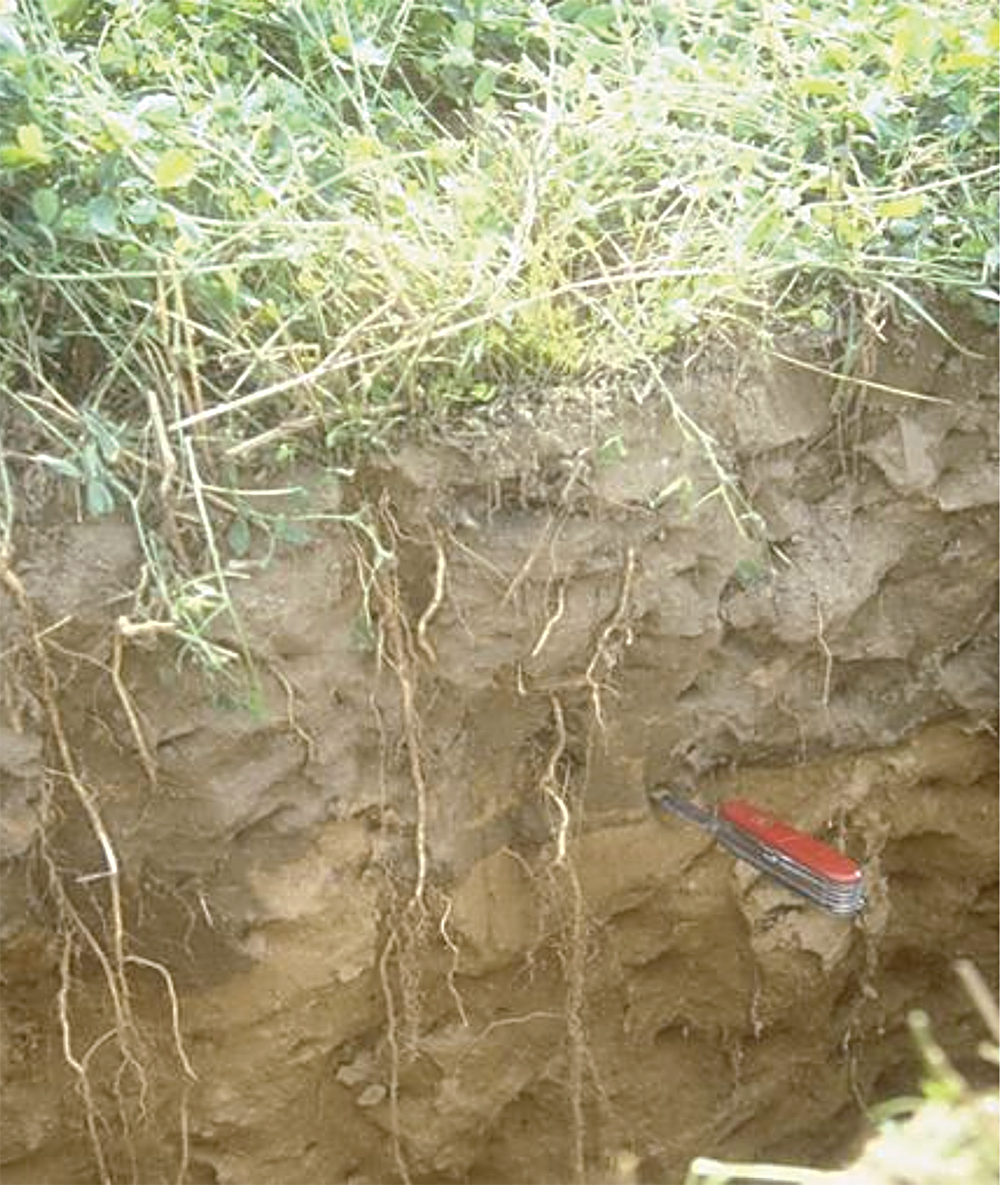
Cover and rotation crops. Cover and rotation crops can significantly reduce soil compaction by creating and stabilizing large voids in the soil that allow for better water and air movement, and by supplying food for microbes. The choice of crop should be determined by the climate, cropping system, nutrient needs and the type of soil compaction. Perennial and cool-season crops commonly have active root growth early in the growing season and can reach into the compacted layers when they are still wet and relatively soft. Grasses generally have shallow, dense, fibrous root systems that have a very beneficial effect by alleviating compaction in the surface layer, but these shallow-rooting crops don’t help ameliorate subsoil compaction. Crops with deep taproots, such as alfalfa, have fewer roots at the surface, but the taproots can penetrate into a compacted subsoil. Forage radish roots can penetrate deeply and form vertical “drill” holes in the soil (see Figure 10.6), as described and shown in Chapter 10. In many cases, a combination of cover crops with shallow and deep rooting systems is preferred (Figure 15.9). Ideally, such crops are part of the rotational cropping system, as is typically used on ruminant livestock farms.
The relative benefits of incorporating or mulching a cover or rotation crop are site specific. Incorporation through tillage loosens the soil, which may be beneficial if the soil has been heavily trafficked. This would be the case with a sod crop that was actively managed for forage production, sometimes with traffic under relatively wet conditions. Incorporation through tillage also encourages rapid nitrogen mineralization. Compared to plowing down a sod crop, cutting and mulching in a no-till or zone-till system reduces nutrient availability and does not loosen the soil. But a heavy protective mat at the soil surface provides some weed control and better water infiltration and retention. Some farmers have been successful with cut-and-mulch systems involving aggressive, tall cover or rotation crops, such as rye and sudangrass.
Addition of organic materials. Regular additions of animal manure, compost or sewage sludge benefit the surface soil layer onto which these materials are applied by providing a source of organic matter and glues for aggregation. The long-term benefits of applying these materials for addressing soil compaction may be very favorable, but in some cases the application procedure itself is a major cause of compaction. Livestock-based farms in humid regions often apply manure using heavy spreaders (sometimes with poor load distribution) on wet or marginally dry soils, which results in severe compaction of both the surface layer and the subsoil. In general, the addition of organic materials should be done with care to obtain the biological and chemical benefits while not aggravating compaction problems.
Controlled traffic and permanent beds. One of the most promising practices for reducing soil compaction is the use of controlled traffic lanes, in which all field operations are limited to the same lanes, thereby preventing compaction in all other areas. The primary benefit of controlled traffic is the lack of compaction for most of the field at the expense of narrow lanes that receive all the compaction. Because the degree of soil compaction doesn’t necessarily worsen with each equipment pass (most of the compaction occurs with the heaviest loading and does not greatly increase beyond it), damage in the traffic lanes is not much more severe than that occurring on the whole field in a system with uncontrolled traffic. Controlled traffic lanes may actually have an advantage in that the consolidated (firmer) soil better facilitates field traffic. Compaction also can be reduced significantly by guiding traffic of farm trucks along the field boundaries and by using planned access roads, rather than allowing them to randomly travel over the field.
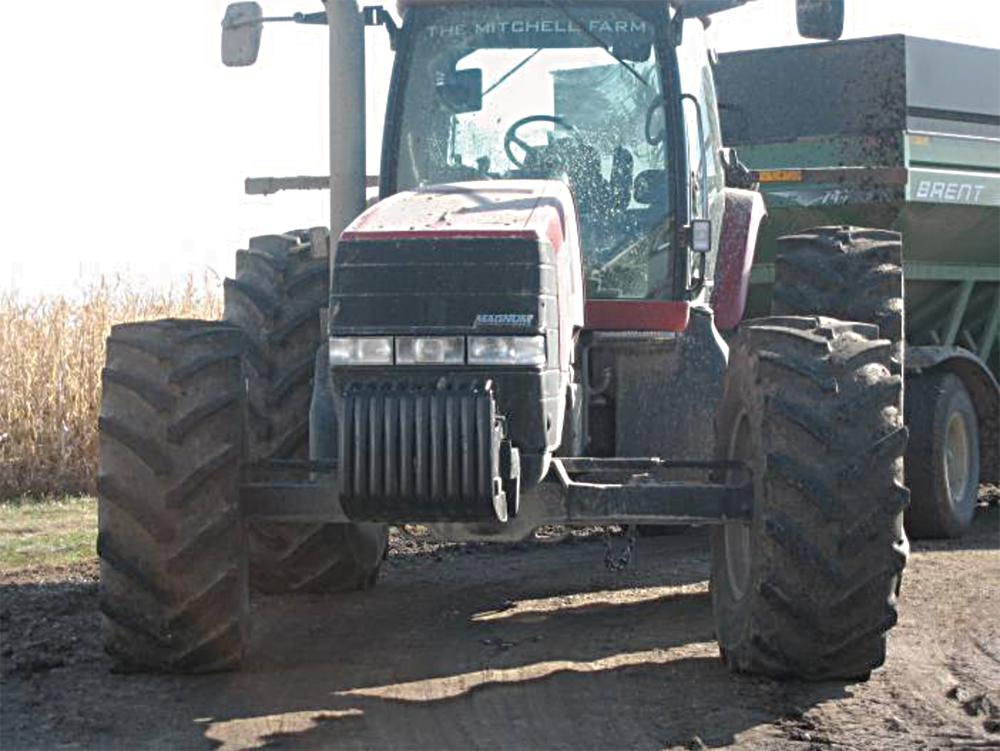
Controlled traffic systems require adjustment of field equipment to ensure that all wheels travel in the same lanes, and they require discipline from equipment operators. For example, planter and combine widths need to be compatible (although not necessarily the same), and wheel spacing may need to be expanded (Figure 15.10). A controlled traffic system is most easily adopted with row crops in zone, strip or no-till systems (not requiring full-field tillage; see Chapter 16) because crop rows and traffic lanes remain recognizable year after year.
Adoption of controlled traffic has expanded in recent years with the availability of RTK (real-time kinematic) satellite navigation systems and auto-steer technology. With these advanced guidance systems, a single reference station on the farm provides the real-time corrections to high levels of accuracy, facilitating precision steering of field equipment. Controlled traffic lanes can therefore be laid out with unprecedented accuracy, and water (for example, drip irrigation) and nutrients can be applied at precise distances from the crop (Figure 15.11).
Ridge tillage dictates controlled traffic, as wheels should not cross the ridges. A permanent (raised) bed system is a variation on controlling traffic in which soil shaping is additionally applied to improve the physical conditions in the beds (Figure 15.11, right). Beds do not receive traffic after they’ve been formed. This system is especially attractive where traffic on wet soil is difficult to avoid (for example, with certain fresh-market vegetable crops, or rice production) and where it is useful to install equipment, such as irrigation lines, for multiple years.
Chapter 15 Summary
Compaction frequently goes unrecognized, but it can result in decreased crop yields or can negatively affect greenery. There are a number of ways to avoid the development of compacted soil, the most important of which is keeping equipment off wet soil. Draining wet soils and using controlled traffic lanes and permanent beds are ways to avoid compaction. Also, reduced tillage and organic matter additions make the surface less susceptible to the breakdown of aggregates and to crust formation, as does maintaining a surface mulch and routinely using cover crops. Reducing compaction once it occurs involves using cover crops that are able to break into subsurface compact layers and using equipment such as subsoilers and zone builders to break up compact subsoil.
Chapter 15 Sources
Gugino, B.K., O.J. Idowu, R.R. Schindelbeck, H.M. van Es, D.W. Wolfe, J.E. Thies, et al. 2007. Cornell Soil Health Assessment Training Manual (Version 1.2). Cornell University: Geneva, NY.
Hoorman, J.J., J.C.M. Sa and R. Reeder. 2011. The biology of soil compaction. Leading Edge 30: 583–587. Soc. Exploration Geophysicists.
Kok, H., R.K. Taylor, R.E. Lamond and S. Kessen. 1996. Soil Compaction: Problems and Solutions. Cooperative Extension Service Publication AF 115. Kansas State University: Manhattan, KS.
Moebius, B.N., H.M. van Es, J.O. Idowu, R.R. Schindelbeck, D.J. Clune, D.W. Wolfe, G.S. Abawi, J.E. Thies, B.K. Gugino and R. Lucey. 2008. Long-term removal of maize residue for bioenergy: Will it affect soil quality? Soil Science Society of America Journal 72: 960–969. Ontario Ministry of Agriculture, Food, and Rural Affairs. 1997. Soil Management. Best Management Practices Series. Available from the Ontario Federation of Agriculture, Toronto, Ontario, Canada.