With these key concepts as the foundation, the goal of systems research is to develop knowledge about how a complex system functions as a whole. This goal, with the assumption that the interactions among components must be studied in order to understand the whole system, is the hallmark of systems thinking. Hence, agricultural systems research strives to develop knowledge about (1) how an agricultural system is influenced by the relationships among its component parts and (2) how that complex system functions as a whole.
From Reductionist Research to Systems Theory: Getting from There to Here
Unlike reductionist research, systems research strives to provide an understanding of how complex systems function as a whole and presumes that (1) a complex system is characterized by nonlinear interactions among its components, and that (2) these interactions create the feedbacks that are the basis for the self-regulatory and emergent qualities of complex systems. In other words, any complex system is more than the sum of its parts. This approach differs from the guiding assumptions of reductionist science, which are that (1) systems can be broken down into their individual components and analyzed as independent entities, and (2) components can be added together in a linear fashion to describe the total system.
"Systems thinking" is a useful approach to organizing information in ways to help understand the complex systems that make up our world.
While reductionist science has a place in the research toolbox, systems-based research, and specifically interdisciplinary systems research (discussed further in chapter 2), provides an additional tool for better understanding real-world complexity while emphasizing the connections between production systems and the associated environmental and social systems.
The roots of agricultural systems research can be traced back to the 1800s, when the first long-term crop rotation studies were established to compare distinct cropping systems. In the United States, most of these early experiments, such as the Morrow Plots (established in 1876 at the University of Illinois, Urbana-Champaign) and Sanborn Field (established in 1888 at the University of Missouri), were initiated to compare simplified rotations with traditional, complex rotations using animal and green manures.
As research shifted in the 1940s to management strategies such as soluble fertilizers, herbicides and pesticides that were effective in much shorter time frames, and therefore could be studied using reductionist methods, cropping systems research fell out of favor.
However, farming systems research, which focuses on economic goals as well as production systems, arose in international development circles during the 1970s as a strategy to help limited-resource farmers improve production technologies (Shaner, 1982). This approach garnered attention from international agriculture and rural development organizations for its promise to overcome the limitations of conventional agricultural research, which was oriented exclusively toward supporting national production and development goals without regard to their effects on rural producers and consumers. For the first time, research teams that included agronomists, economists and farmers began to study working farms. By the late 1980s, this type of research had become institutionalized in national and international agriculture research organizations worldwide (Baker and Norman, 1990).
In the United States, interest in whole-systems research was revived in the 1980s, largely as a result of the sustainable agriculture movement. Much of the early research around sustainability drew from the international farming system movement. The Farming Systems Trial at the Rodale Institute was the earliest of these second-generation cropping system experiments in the United States (Liebhardt et al., 1989; Peterson et al., 1999). This long-term trial influenced numerous cropping systems experiments established in the United States during the 1980s and 1990s. Early examples funded by SARE include the Sustainable Agriculture Farming Systems (SAFS) Project in Davis, California, (see p. 35), the Research Unit at the Center for Environmental Farming Systems (CEFS) in Goldsboro, North Carolina (see p. 20), studies on forage and livestock systems in High Plains agriculture in Texas, and a study on transitioning from conventional to organic agriculture in West Virginia. More recent examples of SARE-funded systems projects include the development of sustainable cropping systems for dairy operations at Penn State and a fully integrated, self-sustaining dairy farm at the University of New Hampshire (see p. 76).
At the same time, ecologists were beginning to apply ideas about ecosystems to agriculture, eventually giving rise to the concept of “agroecosystems” and a new conceptual framework for studying agricultural systems known as agroecology (Gliessman, 2014). By providing a solid theoretical and conceptual base, agroecology has played a huge role in the development of whole-systems thinking in agriculture (Gliessman, 2014). Much of the theory and many of the ideas presented in this handbook are derived from agroecology and agroecosystem-based research.
While systems-based research cannot replace reductionist science, it can provide an additional tool to better understand the complexity of the real world while emphasizing the connections between production systems and the associated environmental and social systems.
Changing Paradigms for Agricultural Research
Moving from a reductionist approach to a systems approach is a complex process that involves more than minor modifications to experimental designs or research protocols. Effective implementation of systems research requires thinking and acting in entirely different ways about agriculture and agricultural systems, scientific collaboration and experimental design.
This shift can be loosely divided into the following three areas:
Rethink. Researchers must move from concentrating on individual components and simplistic cause-and-effect relationships, to a holistic view that encompasses all parts of the system and the interconnectedness among those parts. In other words, researchers must think in terms of whole systems when defining the system under study.
Redesign. Researchers must overhaul the way in which they design agricultural research as they move from factorial to systems approaches.
Regroup. Collaboration is essential. Because real-world agronomic problems rarely occur within disciplinary boundaries, teams of scientists and agricultural practitioners who are familiar with different parts of the system and who can share their expert knowledge must work together to accomplish problem solving. In many large-scale systems research projects, particularly those funded by SARE, farmers are at the research table from day one, providing input and suggestions for design and implementation.
Rethinking the Experimental Approach
The move away from a focus on individual parts and cause–effect relationships to a holistic view is critical to applying a systems approach. A good first step is to develop a conceptual model or map of the components and interactions that make up the system of interest. Such models can be very useful in developing the research questions and hypotheses. For example, consider the following case study that contrasts reductionist and systems approaches to management of the soybean aphid.
Researchers following a conventional reductionist approach would likely address an aphid problem by asking, “How can the soybean aphid be controlled?” They might then design a short-term factorial experiment to test specific practices, such as varying rates and types of pesticides, working from a reductionist position that soybean aphid dynamics are independent of other factors in the agricultural environment.
Researchers using a systems-based approach would begin differently, perhaps by framing the question in broader terms by asking, “What interactions and processes within the agricultural system regulate/impact soybean aphid populations and life cycle?” Because so many possibilities exist, a practical next step might be to represent the options graphically using illustrations or a concept map.
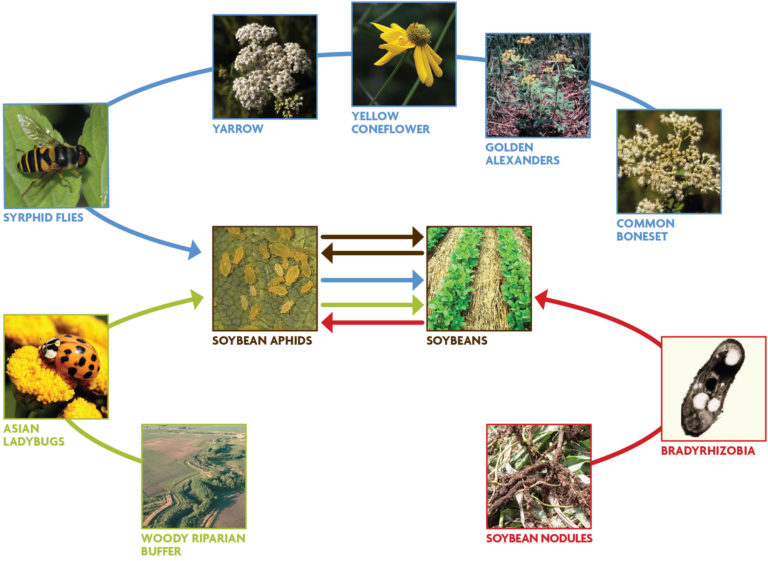
Figure 1.1 is an example of a concept map that could be used by a collaborative research team; the map shows processes and interactions that could affect soybean aphid populations in a Midwest corn–soybean system. The red arrow indicates naturally occurring strains of bradyrhizobia, nitrogen-fixing bacteria that inhabit soybean nodules and that confer resistance to soybean aphids (Dean et al., 2009). The blue arrows point to native plant species that provide habitat for syrphid flies, a natural enemy of soybean aphids (Rutledge et al., 2004). The green arrows indicate the Asian ladybug, also a natural enemy of aphids. Asian ladybugs overwinter in trees and tend to be abundant in regions with woody buffers (Gardiner et al., 2009).
This concept map allows researchers to view known interactions that could contribute to aphid control, thus helping them shape the direction of their research. This example demonstrates that systems research entails painting a more expansive and interconnected picture than traditional reductionist methods. It also suggests that a systems approach to research and management of soybean aphids (and other insect pests) involves the use of multiple strategies rather than a single, “silver bullet” solution. (See chapter 2 for more information on the use of concept maps.)
Redesign
The most commonly used approach in standard agricultural research is the factorial design. In a factorial design, researchers simultaneously study the effects of two or more factors at two or more levels, usually by comparing various combinations (Little and Hills, 1978), while holding all other attributes constant. This design allows investigators to deconstruct a complex system, isolate specific components and identify cause-and-effect relationships about production questions. For example, a factorial design approach could be used to ask, “How do specific tomato varieties respond to varying rates of organic or inorganic fertilizer?” This research would evaluate the response of each tomato variety to organic and inorganic fertilizer rates in isolation of other factors.
Box 1.2: Challenges of Reductionist Design
Researchers using factorial designs face a number of challenges, one of which is the fundamental assumption that experiments accurately simulate the agricultural system under study. In many instances, experimental designs aimed at isolating specific processes or factors result in oversimplification; in an effort to hold all variables constant except for those being tested, investigators change—or even completely eliminate—key interactions among parts of the system. For example, when researchers test organic farming systems by varying one factor in isolation—such as by comparing plots that have been sprayed with herbicides to plots with mechanical weed cultivation—the organic systems may not appear to perform as well as conventional growing systems because management history has changed the background conditions (e.g., soil properties and weed populations), and specific adjustments (e.g., to rotation, tillage, planting date) must be made to compensate for the absence of chemical weed controls. To study organic farming systems properly, a systems-based experimental design that includes all of these parts must be used.
Factorial designs can also lead to the use of experimental plots in which management practices do not reflect a realistic production system. To minimize variation across treatments, factorial design dictates that all management practices other than experimental factors remain the same for all treatments. In the real world, however, farm management systems are interconnected and a change in one practice usually means that other components must be modified. For example, changes in tillage often necessitate shifts in planting date or crop variety. Such shifts contribute to the challenges of using factorial designs and highlight another advantage of systems-based research: it is generally more compatible with the way real farmers approach management and problem solving.
However, in reality, tomato plants respond to many factors other than the type or rate of fertilizer, such as soil structure, moisture and pest pressure. Because factorial designs do not simulate a system, but instead isolate specific processes or factors, they often result in oversimplification. In an effort to hold all variables constant except for the factors being tested, investigators change or even eliminate key interactions among parts of the system (Drinkwater, 2002) (see Box 1.2).
A common mistake in many early agricultural systems research projects was to apply this factorial approach to new systems under development. This led to poor results, because the experimental design simply represented a modified reductionist approach (Janke et al., 1991). Rather than redesigning the system to include the many factors that are essential to the success of a sustainable system—soil health, microbial diversity, cover crops, economic price advantages and so on—researchers simply removed and/or substituted one factor at a time, such as herbicides or pesticides. To truly test an entire system, the design must allow each management regime or treatment to perform optimally, even if this means widening the variability in production practices and experimental design.
An excellent example of design that mimics a system, rather than substituting practices, is the Sustainable Agriculture Farming Systems (SAFS) experiment in Davis, California. A SARE-funded project that ran for many years, SAFS (see p. 35) was a long-term trial that compared conventional and cover cropped tomato rotations. Early in the experiment, farmer cooperators pointed out that the cover cropped system was performing poorly because it was planted late due to the need to plow down fertility-providing cover crops. So the research team made an adjustment: they modified the system to fit typical best farming practices in the area by switching to tomato transplants in the cover crop system, rather than mimicking the conventional system. This meant the cover cropped system had a later planting date, used different varieties and had a completely different irrigation schedule. After these modifications were made, the cover cropped system became not only economically competitive, but also more efficient in terms of energy and water use. Had the researchers held planting dates and varieties constant between the two systems, the cover cropped system would likely never have been economically competitive and its potential performance would have been severely underestimated.
This example also points to the need for longer time frames to reap the full benefits of agricultural systems research. First, researchers need time to gain experience and to adjust the experimental design, even if they have had early input from farmers. Second, short-term experiments that typically last only two to three years provide little opportunity to analyze ecological processes that may take many years to manifest. For example, soil nutrient availability is influenced by soil management history, and many soil processes require far more than one or two growing seasons to approach a steady state. Specifically, nitrogen availability is determined by a combination of very rapid processes, such as microbial biomass turnover, and slower processes that regulate the quantity and composition of soil organic matter (SOM). As a result, when soil treatments such as no-till are introduced after decades of conventional tillage, changes in nitrogen and carbon cycling can reduce plant-available nitrogen for the first few years (Meisinger et al., 1985). This effect declines over time, however, as SOM reaches a new steady state. Similarly, shifting from inorganic nitrogen fertilizers to organic amendments such as compost or green manures also initially reduces nitrogen availability, which then increases gradually as soil-cycling processes reach a new steady state (Liebhardt et al., 1989; Clark et al., 1999). If trials are designed to reflect only short-term effects such as yield response, they cannot accurately measure the effects of biological processes that take considerably longer to manifest.
Systems research redesign also means that larger study sites or plots than those typically used in standard reductionist research trials are required. Landscape characteristics at scales larger than common experiment station plots have been shown to influence experimental outcomes such as crop damage (Letourneau, 1997) and pest abundance, suggesting that in some cases, it may be necessary to move beyond the experiment station to include surrounding areas in the research. For example, large-scale research has shown a link between landscape-level vegetation and the populations, diversity and behavior of beneficial insects, including natural enemies such as parasitoids. In a study by Marino and Landis (1996), the diversity of landscape-level, not field-scale, vegetation determined the effectiveness of parasitoids on armyworm larval stages in maize fields. Although parasitoid species diversity was similar at both landscape scales, mean percentage parasitism was significantly higher in fields situated in a complex landscape compared to fields surrounded by a simple landscape (13.1 percent versus 2.4 percent). Such findings suggest that replicated factorial experiments conducted in small plots at single locations are not always adequate for evaluating the efficacy of biological pest control practices.
Systems redesign presents a particular challenge in that not only must each system be defined and evaluated differently (see chapters 3 and 4), systems studies also generally include a variety of experimental designs and often use multiple strategies within a project. These strategies can include on-farm and participatory research, case studies, surveys and interviews, focus groups, landscape-level data collection and differing management regimes.
Finally, while systems-based research emphasizes the study of multiple components and their interactions, it does not preclude the use of tools that are also used in reductionist approaches, such as replication, factorial design and statistical analysis. Controlled experiments that can identify cause-and-effect relationships and pinpoint underlying mechanisms are useful for solving complex problems within a systems context. For example, studies of long-term farming systems often use a randomized complete-block design with a cropping system as the main treatment (Liebhardt et al., 1989). Smaller, short-term factorial experiments are frequently used as “satellite trials” to test specific practices to be incorporated into larger cropping systems experiments. Investigators also embed smaller replicated plots, sometimes with factorial treatments, in farm fields or large experiments (Kramer et al., 2002; Schipanski et al., 2010). All of these approaches are discussed in more detail in chapters 3 and 4.
Regrouping
By its very nature, systems research is most effective when conducted collaboratively by multi- or interdisciplinary teams that have a large body of collective knowledge. In the example of the soybean aphid (p. 14), the research team pursuing a systems approach would have needed, at a minimum, experts in entomology, plant pathology and soil biology. As problems and the questions developed to address them become more complex, the composition of the team must become more diverse.
Nitrogen loss is an example of a phenomenon or process that can be best understood from a systems perspective using a team with diverse areas of expertise. At the biophysical level, nitrogen loss depends in large part on the amount, timing and type of fertilizer a farmer applies to a field, and much research has focused on optimizing these management factors. However, the farmer’s application rate is not only based on these management considerations but is also influenced by environmental and social factors including soil conditions, crop rotation, government policies, private markets and the farmer’s views about the costs and benefits of fertilizer. Furthermore, once the fertilizer is applied, a wide range of environmental processes such as rainfall patterns and bacterial nitrogen transformations continue to affect the rate of nutrient loss.
From this brief summary, it should be clear why a diverse team is essential to approaching agricultural research from a systems perspective. Agronomists are needed to focus on improving fertilizer use efficiency. Soil nutrient management specialists would research improved fertilizer application methods that enable crops to use a greater proportion of applied nutrients. Extension educators would communicate information about best management practices to farmers. Plant physiologists and molecular geneticists could tackle crop improvement, including the development of crop varieties that use nitrogen more efficiently. Experts in water management and hydrology would explore engineered solutions, such as the construction of swales and wetlands to “soak up” excess nitrogen. Economists and other social scientists would be on board to evaluate human factors.
For example, in the Judith Basin in Montana, biophysical scientists proposed changing the type, amount and rate of fertilizer application to address a nitrate problem, but farmers were not interested in reducing fertilizer rates at the expense of yields. After conducting extensive surveys and interviews with the farmers, a team of sociologists identified the need to reframe the research away from a singular focus on nitrogen fertilizer management to a broader, systems-level effort aimed at acquiring more information on nitrate dynamics in the field and under different management systems. This shifted the emphasis away from identifying fertilizer as the problem to a much broader view of the crop and soil system, which made sense to the farmers. Working together, farmers and scientists reframed the research question and jointly interpreted the results, which showed that the intersection of crop rotation status, soil nitrate levels and rainfall patterns determined when and where nitrate pulses to groundwater were likely to occur. This approach provided a more complex picture of the issue and increased the likelihood that researchers could develop solutions that farmers were apt to use. (See Box 1.3 for other examples of how social scientists have played a key role in systems research projects.)
Box 1.3: Sociology in Systems Research
Eco-friendly, biodegradable alternatives to polyethylene plastic mulch have been available since the 1980s, but vegetable growers have long been reluctant to use them, even though they are cost-effective in the long term, require less labor and are more environmentally sustainable than plastic mulches. To better understand this reluctance, a multidisciplinary team of researchers from Washington State University, the University of Tennessee, and Texas A&M University, with sociologists at the forefront, conducted focus groups and surveys with farmers and extension educators. The group found that farmers were disinclined to use biodegradable plastic mulches because of insufficient knowledge about the technology, high upfront costs, unpredictable breakdown in the soil, and unknown soil impacts. The research, funded by a USDA-SCRI grant from 2009 to 2013, was the first of its kind: it applied sociological research to document the perceptions of vegetable farmers in order to determine barriers and bridges to using alternatives to polyethylene plastic mulch. With this information, the multidisciplinary team is now working beyond the typical field studies that test new mulch products and is working directly with farmers to find biodegradable mulches that will be effective. The team is developing an outreach strategy so that farmers have the opportunity to learn the ins and outs of using the new technology. In this example and in the Judith Basin nitrogen case mentioned on p. 17, the problem was approached at first from a purely environmental perspective, and without success. However, the inclusion of social scientists provided the opportunity for farmers’ voices to be heard. By focusing on farmers as an integral part of the ecosystem and by determining what motivates their decision-making, social scientists enable a team to develop more applicable, lasting solutions. University of Vermont anthropologist Jason Parker notes that understanding the stakeholders in the system is integral when working with a specific community. “It’s not about learning [a specific language] but learning how people speak and think about a particular issue or topic. If you can do that, then you are likely to be more successful collaborating with people to share a project’s outcomes,” says Parker. Sociologists understand that the factors influencing farmers’ decisions are complex and go beyond simple economic or environmental considerations. In fact, because farms are often run as a family unit, they are not traditional businesses, and therefore farmers are not traditional businessmen and women.
Farmers make decisions based on influences at varying societal levels, including the following:
- Individual: farmer, family members, farmworkers
- Household: family resources and needs, land availability, farm succession
- Community: neighborhoods, community groups and organizations
- Institutional: state and government rules, regulations, policies, population’s needs.
Sociologists interpret how farmers interact within these societal levels in the agricultural system. Involvement of a sociologist in a research project leads to a deeper understanding of the farmer’s perspective, which may not be obvious to a biophysical scientist. According to Dr. Shoshanah Inwood, rural sociologist at the University of Vermont, sociologists recognize that farmers are diverse. They make decisions based on different drivers, values and experiences, meaning that similar systems research projects in different regions of the United States might yield totally different results. “When we talk about policy and programs, we can’t assume that all farmers are going to be able to engage in the programs in the same way,” she says. In systems research that aims to address connections between society and production systems, a sociologist should be on the team from day one to help guide the development of the study to effectively explore societal influences on farm management decisions. SARE has found that having sociologists on planning teams is integral to advancing sustainable agriculture because of the critical role the sociologist can play in drawing connections across the biophysical and social divide. Each of SARE’s four Administrative Councils, which help determine the grants that the program will fund each year, has a rural sociologist representative. This representative reviews research proposals to determine if they are asking appropriate questions that factor farmers’ livelihoods into the research approach.
“Changing the food system and sustainability is a three-legged stool. We need crop scientists, we need animal scientists and we also need social scientists. In the long run, this will improve the quality of the food system in the United States,” says Doug Constance, a sociologist and Southern SARE representative.
The importance of including sociologists, economists and other social scientists from the start and throughout the project cannot be overstated. Many of the current environmental problems in agriculture and the failure to produce results that translate into economic security for farmers stem directly from this lack of cross-disciplinary work. Seeking to broaden their approach to understanding postharvest handling of fresh fruits and vegetables from farm to consumer, faculty from the Food Science and Agricultural Engineering departments, as part of the University of Georgia Postharvest Research Team, joined with an agricultural economist to develop a research plan that expanded beyond the physical and quality aspects of postharvest handling. Together, this interdisciplinary team expanded their reach to look at how postharvest handling affected price and to determine what price the market would bear. Had the team not included the economist from the start, it would not have been able to apply hedonic price modeling, i.e., to examine price factors resulting from both internal characteristics and external factors (Box 1.4).
Box 1.4: The Role of Economists in Systems Projects
Often, in typical cross-disciplinary projects, the following scenario occurs: A paper (or grant proposal) based on biophysical research is submitted for review. The research (or plan) is complete and the authors wait for the (hopefully) minor changes suggested by the reviewers. The review comes back and is generally favorable with the exception of one dreaded phrase: “This research is interesting but we suggest adding an economic component to the work.” With that, the principal investigator (PI) looks at the list of faculty in the economics department to see if he or she knows anyone there. Failing that, a call to the department head connects the PI with an economist who might have some interest in the general area. After describing the project, the PI asks if the economist would be interested in working on it. When the economist asks when the project will start, the answer is that the project is actually over, and that “We just need a few budgets run to make sure our results make economic sense for the farmer.” This is the typical way in which economists are involved in multidisciplinary projects—rarely at the beginning (usually at the end), with a request for some budget numbers to prove that the results obtained do not lower profits. Not surprisingly, the PI is usually disappointed with the negative response he or she generally receives. After hearing that “running budgets” is not something economists do regularly as part of our research (getting budgets published is impossible), and being informed that, at best, perhaps a master’s student could help next semester after classes are finished, the PI is still baffled. Economics is budgets, isn’t it? A lack of understanding of the science, as well as the social science, of economics often brings researchers from the physical disciplines and economists to a standstill. But consider an alternative scenario in which an interdisciplinary team is formed to explore an agricultural system. That system can be large (from farm to consumer) or it can be some subset that crosses boundaries in the production and distribution process. Given that the system involves multiple subsystems and components, it makes sense to look at how economic incentives affect decision-making. From the beginning of the project, the interdisciplinary team includes an economist so that when plans are formed and a research protocol is established, the economic component of the system is included in the objectives and methods. Budgets may be a small part of the effort, but the project will address an important economic question that would not have been asked if the economist had not been involved at the outset. Most important, the project design will include gathering economic data at the same time as other data, which will allow the economic component to fully match the physical aspects of the research. The Postharvest Research Team at the University of Georgia operated in such a manner when investigating how fresh fruits and vegetables were handled from farm to consumer. We were careful to make sure that in each of our projects, all three researchers could identify a research product that would be publishable in journals within our disciplines as well as in interdisciplinary outlets. We shared coauthorship of all work (the author order depended on the publication) and planned all data gathering and analysis with the three disciplines in mind. Successful interdisciplinary research is difficult; meetings are often time consuming, understanding the research needs of other disciplines can be challenging, and gathering all the necessary data can be expensive. Yet, there is no substitute for interdisciplinary research in order to fully understand systems and to perform insightful systems research. The most interesting and useful research I have done has been with colleagues in those other disciplines—and we never did a budget.
Jeff Jordan, Professor of Agricultural and Applied Economics University of Georgia
Along with including multiple research disciplines, agricultural systems research also requires expansion beyond academia. Research is not created in a vacuum; it stems from and reflects current farming practices. Whereas traditional research paradigms have tended to assume a linear relationship with one-way flow—researchers develop innovations, extension workers recommend and spread them, and farmers adopt or reject them—in reality, interactions and knowledge flows among farmers, extension educators and researchers are multidirectional.
Because agricultural systems research is designed to incorporate multiple views and approaches, it widens the scope of who is considered an expert by valuing different ways of knowing, thus opening the research table to multiple stakeholders: extension, community groups, government agencies and farmers—the very practitioners who are the recipients of the research. Agricultural systems approaches can more easily involve farmers and other stakeholders at all stages of the research process—initiation, planning and implementation— and enable all participants to learn from each other. By improving the flow of information from the start, agricultural systems research also encourages new kinds of innovation. Chapter 2 discusses regrouping and collaborative team building.
The case study from the Center for Environmental Farming Systems in North Carolina demonstrates how multiple stakeholders can use all of these approaches—rethinking, redesigning and regrouping—to find new solutions to old problems.